Application of piezoelectric nanogenerator in medicine: bio-experiment and theoretical exploration
Introduction
Implantable and wearable medical electronic devices such as cardiac pacemakers, implantable cardioverter defibrillators (ICD), left ventricular assist devices (LVAD), and total artificial hearts (TAH), etc. are more and more widely used in medicine. They can allow in situ, real-time biomedical detection, monitoring and treatment. However, traditional lithium batteries with limited electric power storage capacity cannot satisfy the function expansion of these devices. When the battery exhausts, patients have to receive surgical operations again to replace the battery of a device, causing the patients’ physical and mental pain and the huge waste of medical resources (1). We hope that one day medical devices can be self-powered without batteries. A living human body produces kinetic energy (from its heart beating, joint movement, muscle stretching, blood vessel contraction and blood flow) and chemical energy (from glucose), etc. These power sources are inexhaustible and non-polluting. The key point is how to convert them into electric energy efficiently.
Zhong-Lin Wang has reported an approach to convert kinetic energy into electric power with the use of aligned zinc oxide (ZnO) nanowires (2). Its mechanism is the combination of semiconducting and piezoelectric nature of ZnO as well as the Schottky barrier formed between the metal and ZnO contacts (3-6). Piezoelectric nanogenerator belongs to the flexible electronic materials, thin and soft, and can convert kinetic energy from muscle contraction and joints movement to electric energy output (7,8). That is to say it has the potential for harvesting mechanical energy from the environment for self-powered electronic medical devices. Our experiment tried to explore if a piezoelectric nanogenerator can be a stable and reliable energy source for implantable electronic medical devices.
Materials and methods
Rectangular piezoelectric nanogenerators (9,10) were provided by Engineering Mechanics and Materials Lab of Tsinghua University (Figure 1). We have performed five animal implants, at the first and second animal implants we tested the nanogenerator at the open chest condition, at the other animal implants we tested the nanogenerator at the closed chest condition. We used Chinese miniature pigs of 30 kg weight with the Guide for the Care and Use of Laboratory Animals published by the National Institutes of Health, prepared with general anesthesia, supine position, median thoracotomy, suspended pericardium and exposed the heart. Then we fixed two opposite edges of a rectangular piezoelectric nanogenerator with 4-0 Prolene (ETHICON) on the surface of the heart at the following positions of the heart (according to the movement style of the heart) (11) respectively to detect the voltage output:
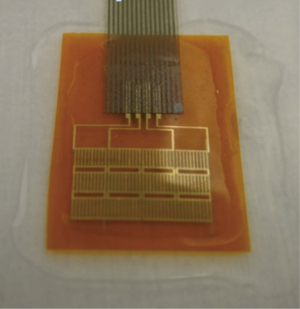
- Position A, right ventricular surface, near the atrioventricular groove, parallel to the long axis of the heart (Figure 2);
- Position B, right ventricular surface, parallel to the atrioventricular groove (Figure 3);
- Position C, left ventricular surface, near cardiac apex, parallel to the left anterior descending branch (Figure 4).
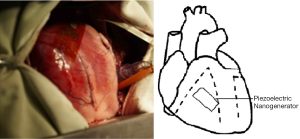
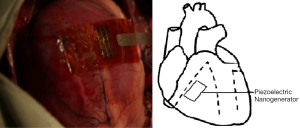
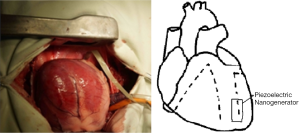
Then we selected the place which has the highest voltage output to fix both ends of the nanogenerator and closed the chest of pig. We recorded the voltage output of nanogenerator under closed chest condition (natural condition) and compared the result with open chest condition (Figure 5). Finally we used Dopamine (positive inotropic agents) and Esmolol (negative inotropic agents) respectively to detect the relation between voltage output of nanogenerator and myocardial contractility.
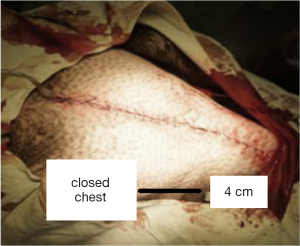
Results
With its both ends fixed on the surface of the heart, a piezoelectric nanogenerator can produce stable voltage output from the contraction and relaxation kinetics of the heart. The piezoelectric nanogenerator fixed on the surface of the right ventricle, near atrioventricular groove, parallel to the long axis direction of the heart produced the highest voltage output stably (3.1 V), compared with those fixed on the surface of right ventricle, parallel to the atrioventricular groove or on the surface of left ventricle, near cardiac apex, parallel to the left anterior descending branch (Figure 6). The voltage is enough for the pacemaker’s operation. The voltage output of the piezoelectric nanogenerator in the natural condition (closed chest) is the same as that in the open chest condition and can make a light emitting diod (LED) light continue to shine, which further confirmed its clinical application value (Figure 7). The voltage output of piezoelectric nanogenerator is positively correlated with myocardial contractile force. The voltage output increased after we used positive inotropic agents (Figure 8) and decreased after we used negative inotropic agents (Figure 9). Experiment process is shown in Figure 10.
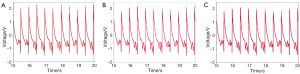
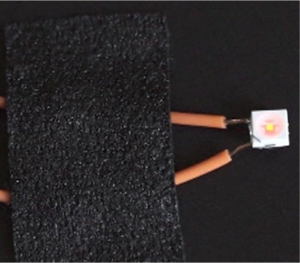
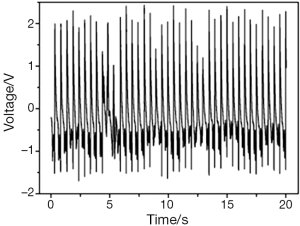
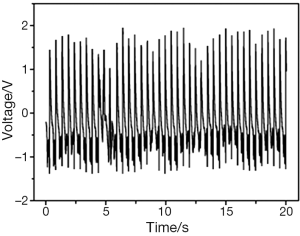
Discussion
Wearable and implantable electronic medical devices such as pacemakers, heart rate monitors, ICD, implantable drug pumps, and brain pacemaker play an important role in continuous clinic and family diagnostics and treatment. Although these electronic medical devices have huge potential application value in medicine, the biggest limitation of them is operational lifetime due to the limitation of battery storage, which rarely exceeds five years for implants and five days for wearable devices. Surgical procedures have to be done to replace the exhausted batteries of implantable medical devices every few years, which may increase the patients’ operative complications, morbidity and potential mortality. To minimize the necessity for surgical operations and contain the medical costs, we try to find alternative ways to overcome batteries’ limitations.
Zurbuchen reported that mass imbalance oscillation generator could be an energy harvester to convert cardiac wall motion to electrical energy (12), but the device was too big for clinical application. John A. Rogers and his team reported that piezoelectric nanogenerators could produce significant electrical power from motions of internal organs (10). The human’s kinetic energy can be converted into electric energy by with the help of piezoelectric ZnO nanowire arrays (13-15). This method has the ability of converting mechanical, hydraulic and vibrational energy into electricity to power electronic medical devices and to monitor physical signs. ZnO combines the properties of piezoelectric and semiconducting. When it is bended, ZnO can create a strain field and charge separation across the nanowire which leads to electric current generation.
The piezoelectric nanogenerator used in this experiment was provided by Xue Feng et al. from Tsinghua University. The nanogenerator is a kind of slice with length of 5 cm, width of 2 cm and thickness of 7.5 µm, combined with piezoelectric materials and micro storage battery. The slice is conformal and can be bended and stretched within certain limits, it can produce voltage output as it deforms and the deformation extent and the voltage output are positively correlated. We encapsulated its surface to avoid corrosion from the body fluid and to minimize its influence on the body’s internal environment.
Our experiment shows that a piezoelectric nanogenerator with its both ends fixed on the surface of the heart can convert kinetic energy from heart beat to electric energy stably. From the comparison of the results from three different positions, we find that the piezoelectric nanogenerator output the highest voltage on the surface of right ventricular, near atrioventricular groove and parallel to long axis of the heart.
Shapes and contraction patterns of the muscles of the left ventricular wall and right ventricular wall resulted in different deformations of the piezoelectric nanogenerator, hence different electric voltage output. The left ventricular wall is generally 9-11 mm thick is thicker than right ventricular wall (3-5 mm). It is roughly cylindrical and contracts in a torsional or twisting pattern with three spiraling layers of wall muscles, it ejects blood by reducing its diameter and circumference whereas wall thickening (16). The right ventricular wall is generally 3-5 mm thick and is wedge shaped with a concave free wall attached to the convex interventricular septum (17) and ejects blood by contracting its free wall (18). The contraction pattern of right ventricular by shortening the free wall results in enhanced wall motion and increased bending of the piezoelectric nanogenerator than the twisting contraction pattern of the left ventricular. At the same time, the longitudinal deformation amplitude of right ventricular free wall is greater than the transverse deformation amplitude, so the position at right ventricular free wall and parallel to the heart long axis (Position A) has the highest voltage output (3.1 V).
Further experiments shows that the voltage output of piezoelectric nanogenerator is uninfluenced under closed chest (natural) condition and can keep an LED light steadily glowing. The voltage produced by nanogenerator can meet the needs of most implantable and wearable medical electronic devices. This discovery provides a new sustainable and non-polluting energy source for medical electronics and make them more miniaturization which will reduce the patients’ wounds eventually.
Besides generating electric energy to power the medical devices, piezoelectric nanogenerators can also be a kind of monitor to cardiac contraction force, heart rate, blood pressure (19), breathing state, diaphragmatic muscle movement, etc. as its voltage output is positively correlated with muscle contraction force and motion state (20). For example, we can make piezoelectric nanogenerator in the shape of a conductor and combine it with pacing leads so that surgeons can monitor cardiac function directly while pacing the heart. At present, doctors can only monitor cardiac function by Swan-ganz catheter or cardiac ultrasound indirectly. In the future, we also can realize accurate, noninvasive and real time arterial pressure monitor by putting the piezoelectric nanogenerator on the surface of the skin (21). With the help of computer and three dimensional (3D)-printing, we can even build the mechanical model of cardiac contraction by nanogenerator to provide guidance in ventricular reconstruction surgery.
In our next step, we plan to test if piezoelectric nanogenerator itself will affect cardiac contraction and its tissue compatibility, we will implant our nanogenerator in the chest and on the surface of heart, lung, diaphragm of pig at least 6 months (chronic animal implant) to test the efficacy, security and compatibility of nanogenerator. In summary, with the development of material science, flexible electronics such as piezoelectric nanogenerator will play a more and more important role in clinical diagnosis and treatment, and they will further reduce patients’ pain and provide convenience to the doctors.
Conclusions
Piezoelectric nanogenerator can convert kinetic energy from contraction of muscle to electrical energy for implantable electronic medical devices. Piezoelectric nanogenerators can output steady voltage on the surface of right ventricle, near atrioventricular groove, parallel to the long axis direction of the heart, which can be the new potential energy source for pacemakers. Piezoelectric nanogeneratosr can be used as cardiac function monitor in the future for its voltage output is positively correlated with myocardial contractile force.
Acknowledgements
Piezoelectric nanogenerator was provided by Professor Xue Feng and Bing-Wei Lu from Engineering Mechanics and Materials of Tsinghua University. The project was supported by “International Science & Technology Cooperation Program of China” from BSTC (2012DFA31110) and “The Standard Diagnosis and Treatment and Key Technology Research of Aortic Dissection” from The Research Special Fund for Public Welfare Industry of Health (201402009).
Disclosure: The authors declare no conflict of interest.
References
- Koutentakis M, Siminelakis S, Korantzopoulos P, et al. Surgical management of cardiac implantable electronic device infections. J Thorac Dis 2014;6:S173-9. [PubMed]
- Wang ZL, Song J. Piezoelectric nanogenerators based on zinc oxide nanowire arrays. Science 2006;312:242-6. [PubMed]
- Zhu G, Yang R, Wang S, et al. Flexible high-output nanogenerator based on lateral ZnO nanowire array. Nano Lett 2010;10:3151-5. [PubMed]
- Dagdeviren C, Hwang SW, Su Y, et al. Transient, biocompatible electronics and energy harvesters based on ZnO. Small 2013;9:3398-404. [PubMed]
- Chen X, Xu S, Yao N, et al. 1.6 V nanogenerator for mechanical energy harvesting using PZT nanofibers. Nano Lett 2010;10:2133-7. [PubMed]
- Anton SR, Sodano HA. A review of power harvesting using piezoelectric materials (2003-2006). Smart Mater Struct 2007;16:R1-21.
- Pfenniger A, Jonsson M, Zurbuchen A, et al. Energy harvesting from the cardiovascular system, or how to get a little help from yourself. Ann Biomed Eng 2013;41:2248-63. [PubMed]
- Hu Y, Lin L, Zhang Y, et al. Replacing a battery by a nanogenerator with 20 V output. Adv Mater 2012;24:110-4. [PubMed]
- Feng X, Yang BD, Liu Y, et al. Stretchable ferroelectric nanoribbons with wavy configurations on elastomeric substrates. ACS Nano 2011;5:3326-32. [PubMed]
- Dagdeviren C, Yang BD, Su Y, et al. Conformal piezoelectric energy harvesting and storage from motions of the heart, lung, and diaphragm. Proc Natl Acad Sci U S A 2014;111:1927-32. [PubMed]
- Fritz J, Solaiyappan M, Tandri H, et al. Right ventricle shape and contraction patterns and relation to magnetic resonance imaging findings. J Comput Assist Tomogr 2005;29:725-33. [PubMed]
- Zurbuchen A, Pfenniger A, Stahel A, et al. Energy harvesting from the beating heart by a mass imbalance oscillation generator. Ann Biomed Eng 2013;41:131-41. [PubMed]
- Li Z, Zhu G, Yang R, et al. Muscle-driven in vivo nanogenerator. Adv Mater 2010;22:2534-7. [PubMed]
- Fan FR, Lin L, Zhu G, et al. Transparent triboelectric nanogenerators and self-powered pressure sensors based on micropatterned plastic films. Nano Lett 2012;12:3109-14. [PubMed]
- Pfenniger A, Vogel R, Koch VM, et al. Performance analysis of a miniature turbine generator for intracorporeal energy harvesting. Artif Organs 2014;38:E68-81. [PubMed]
- Rushmer RF. Length-circumference relations of the left ventricle. Circ Res 1955;3:639-44. [PubMed]
- Fritz J, Solaiyappan M, Tandri H, et al. Right ventricle shape and contraction patterns and relation to magnetic resonance imaging findings. J Comput Assist Tomogr 2005;29:725-33. [PubMed]
- Anzola J. Right ventricular contraction. Am J Physiol 1956;184:567-71. [PubMed]
- Pfenniger A, Obrist D, Stahel A, et al. Energy harvesting through arterial wall deformation: design considerations for a magneto-hydrodynamic generator. Med Biol Eng Comput 2013;51:741-55. [PubMed]
- Wang ZL, Wu W. Nanotechnology-enabled energy harvesting for self-powered micro-/nanosystems. Angew Chem Int Ed Engl 2012;51:11700-21. [PubMed]
- Pfenniger A, Wickramarathna LN, Vogel R, et al. Design and realization of an energy harvester using pulsating arterial pressure. Med Eng Phys 2013;35:1256-65. [PubMed]