Ultrasound assessment of upper airway dilator muscle contraction during transcutaneous electrical stimulation in patients with obstructive sleep apnoea
Introduction
Obstructive sleep apnoea (OSA) is the most common form of sleep-disordered breathing (1,2). It represents a global health problem that causes daytime symptoms like sleepiness and affects the cardiovascular system (3,4). Symptomatic OSA affects up to 10% of middle-aged men and 3% of middle-aged women (1,2). Almost a quarter of the 30–69 year-old population in the UK may have mild OSA, while 4.8% of the same age range might have moderate to severe OSA (5).
OSA is characterised by repeated episodes of partial or complete upper airway collapse that results in hypopnoeas or apnoeas while asleep and leads to intermittent hypoxia, frequent arousals and sleep fragmentation which causes daytime symptoms like excessive daytime sleepiness and impaired cognition (6). Several pathophysiological mechanisms such as upper airway anatomy and control, ventilatory control and arousal threshold contribute to OSA, and phenotypes can vary considerably between individuals (7,8). Obesity, male gender, age, alcohol and smoking are potential risk factors for OSA (9-12). Diminished upper airway dilator neuromuscular tone to maintain airway patency during sleep represents a major contributing factor (13,14).
Therapeutical recommendations for OSA include continuous positive airway pressure (CPAP), oral appliances (mandibular advancement device, MAD) (15), positional therapy (16), behavioural interventions, such as lifestyle advice including weight loss, smoking cessation, and reduction of alcohol and sedatives use (17), bariatric surgery (18,19) and in a minority of selected cases upper airway surgery or maxillomandibular advancement surgery (20,21).
CPAP therapy remains the first line therapy for OSA in most cases (22). It acts as a pneumatic splint and delivers pressurised air into the upper airway to maintain patency while asleep (17,23). It improves OSA associated symptoms, quality of life and reduces the clinical sequelae. However, long-term adherence to CPAP remains limited (24,25) and effective second line therapies are needed.
Electrical stimulation (ES) of the upper airway dilator muscles during sleep is a relatively novel approach to treat OSA. It targets the diminished neuromuscular tone of the upper airway dilator muscles, particularly the genioglossus muscle (GG), that promotes upper airway collapsibility (14,26,27). External activation of the dilator muscles can help to maintain airway patency while asleep with different methods employing this approach, invasively and non-invasively (28-31).
Hypoglossal nerve stimulation (HNS) is a unilateral method, approved by the United States Food and Drug Administration (FDA) in 2014 following publication of the STAR trial results (29). The National Institute for Health and Care Excellence (NICE) in the UK published its interventional procedure guidance on HNS in 2017 (32). In a follow up cohort study of patients with HNS it was shown that safety, treatment efficacy, improved sleepiness and quality of life were sustained over five years of use (33). Another invasive approach is the bilateral HNS stimulation using the Genio™ system (Nyxoah SA, Mont-Saint-Guibert, Belgium). It makes use of an implanted neurostimulator system that is activated by a battery-unit worn externally and effectively reduces OSA severity and improves quality of life (34).
As a non-invasive approach, transcutaneous ES in OSA (TESLA) was first introduced in 2011 (35). It is delivered via transcutaneous patches that apply low currents to the submental area throughout the night, stimulating the upper airway dilator muscle group without disturbing sleep (30,35). In a randomised sham-controlled crossover trial, patients significantly improved in the 4% oxygen desaturation index. Responders to the treatment were found to be slimmer, predominantly female and with mild-to-moderate OSA (30). This method is currently undergoing further assessment regarding usability, functionality and task accomplishment in clinical trials (36). A recent meta-analysis of HNS and transcutaneous ES concluded that there was a significant effect size, reducing the severity of OSA, when using ES (37).
Effective assessment of upper airway dilator muscle stimulation and contraction is required to identify potential responders to these methods. However, most methods to visualise the upper airway are cumbersome and require costly equipment (MRI) (38) and time (drug-induced sleep endoscopy, DISE) (39). In contrast, ultrasound is a non-invasive bedside device that is available in most hospitals. It can visualise the tongue in different planes and evaluate the contraction of the upper airway dilator muscles in response to ES (35,36,40).
In the current study, we sought to evaluate the use of ultrasound to demonstrate the contraction of upper airway dilator muscles using transcutaneous ES in patients with OSA while awake.
We present the following article in accordance with the STROBE checklist (available at http://dx.doi.org/10.21037/jtd-cus-2020-001).
Methods
This was a physiological sub-study of a randomised controlled trial (NCT03160456) at Guy’s and St Thomas’ NHS Foundation Trust in London, UK. The trial was conducted in accordance with the Declaration of Helsinki and the Harmonized Tripartite Guideline for Good Clinical Practice from the International Conference on Harmonization. The study included recruited patients from the Lane Fox Unit and the Sleep Disorders Centre between July 2018 until March 2019. Following approval by the research ethics committee (IRAS ID 217448), as well as the NHS Trust’s R&D department, eligible patients received the patient information sheet prior to discussions of eligibility; written and informed consent was obtained in all patients.
Objectives
The main outcome of interest of this sub-study was to determine the increase in tongue base diameters during transcutaneous ES (D2 ‒ D1 = ΔD) measured in mid-sagittal and coronal planes, as well as the increase in the cross-sectional area (CSA) of the tongue base in the coronal view while ES was turned on and off (A2 ‒ A1 = ΔA).
Secondary outcome parameters were to address the best planes and diameters showing muscle contraction during ES (delta and % of change), correlations between measurements in different planes (Pearson correlation analysis), establishing the most feasible method of tongue ultrasound examination in different scanning planes, and classification of the scanned ultrasound pictures based on quality and clarity of the targeted measurements (semi-quantitative score, 0‒2 points).
Inclusion criteria
Patients with mild to mildly severe OSA who were diagnosed using overnight polysomnography with an Apnea-Hypopnea Index (AHI) >5/hour to <35/hour plus symptoms of sleepiness (ESS >10 points), who failed and/or had withdrawn from CPAP, with a body mass index (BMI) of 18.5‒32 kg/m2 were included.
Exclusion criteria
Exclusion criteria were based on our previous experience (TESLA trial) (30), the eligibility criteria of the STAR trial (29) and other trials that used HNS. A range of co-morbidities made patients non-eligible for recruitment into the trial: non-respiratory sleep disorders, relevant respiratory and cardiovascular co-morbidities, facial hair affecting placement of the hydrogel patches, and known allergies to plasters or similar products.
Short protocol
Ultrasound was used to assess the functionality of transcutaneous electrical current to stimulate the upper airway dilator muscles by studying the change in tongue diameters in different scanning planes, in awake patients, seated, with and without the ES turned on. Each patient came for their assessment to Guy’s & St Thomas’ NHS Foundation Trust, the medical history was recorded, and a general physical examination took place, tongue ultrasound examination with and without transcutaneous ES, as well as a polysomnographic sleep study (Alice 6 equipment, Respironics, Murrysville, Pennsylvania, USA) were recorded to establish the patients’ AHI.
Transcutaneous ES and ultrasound examination
The patients were awake and in seated posture with the head supported by the investigator’s non-dominant hand, and a relaxed and slightly opened mouth.
The skin in the patient’s submental area was wiped using alcohol pads. Then, two self-adhesive hydrogel skin patches (4×4 cm2; TENS company, Everway Medical Instruments Co Ltd) were placed in the submental area midway between the angle of the mandible and the chin, as previously described (30,35,36) (Figure 1).
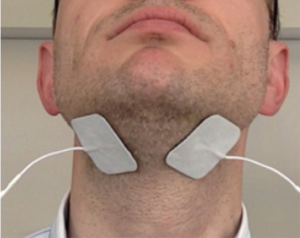
The patches were connected via cables to a transcutaneous ES (TENS) machine (Premier Combo Plus, EM-6300A TENS/EMS, Everway Medical Instruments Co Ltd).
First, the machine remained switched OFF, skin patches were kept in place and the ultrasound scans were obtained (LOGIQTM, GE Healthcare, WI/USA).
GE 9L-RS superficial linear probe (band width 3.33–10 MHz, GE Healthcare, WI/USA) was used for imaging, in frequency 8–10 MHz, and 3.5–5 cm depth (the depth setting differs according to neck circumference as well as the tongue bulk).
A generous amount of ultrasound gel was applied for better ultrasound transduction in the presence of the skin patches.
The transducer was positioned 2 cm from the chin in the mid-sagittal plane (Plane A), pointing cranially. Scans in this plane showed the skin, subcutaneous tissue and the thickness of tongue base muscles. The patient was then asked to move the tongue to confirm its’ visualization in the real-time sonography. Repeated images were collected and saved for offline measurements and analysis (Figure 2).
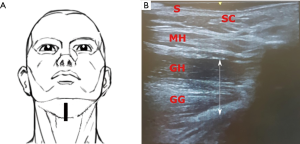
In a next step, the probe was turned 90° pointing cranially to scan images in coronal view (Plane B). This view showed the skin, subcutaneous tissue, the two anterior bellies of digastric muscles, mylohyoid, geniohyoid and genioglossus muscles. Images in this plane were saved (Figure 3).
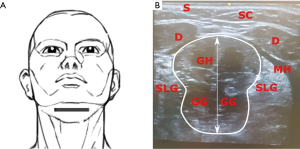
Ultrasound scans in both planes were performed again during transcutaneous ES. The electrical current was delivered at 30Hz and pulse duration of 250 µs. The current required to evoke a visible muscle contraction differed among patients.
Positions of the probe for each scanning plane are kept unchanged. The distance between the upper margin of the geniohyoid muscle to the lower margin of the genioglossus muscle represents the tongue thickness. This distance with stimulation (D2) and without stimulation (D1) allowed to calculate the change in diameters (D2 ‒ D1 = ΔD). The increase in the CSA of the tongue in the coronal plane is represented as (A2 ‒ A1 = ΔA) (Figures 4,5).
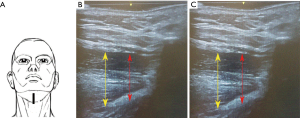
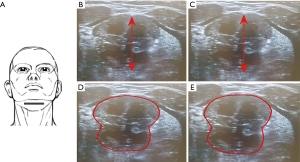
Offline evaluation of the scans regarding picture quality using a semi-quantitative scale (0–2 points, Figure 6), with ‘0’ indicating poor and ‘2’ indicating the best quality:
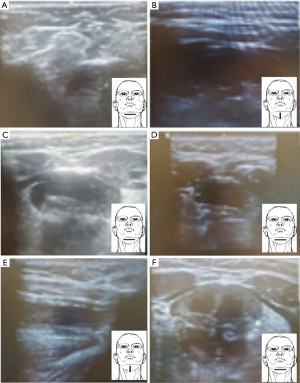
❖ Poor quality score (‘0’), scans could not be clearly assessed due to blurred pictures, unadjusted and/or uncentralised view, not showing the structures of interest, unadjusted depth.
❖ Acceptable quality score (‘1’), scans were of good average picture quality and resolution, but some of the structures were difficult to identify.
❖ Good quality score (‘2’), scans could clearly assess and record all structures and measurements in both planes, no blurring with distinct borders, good view, with stimulation on and off.
Offline data analysis
At least four different pictures for each plane were recorded and assessed for quality (0–2 points), on and off stimulation, respectively. Each structure was measured at least three different times on each picture, the average of these measurements was recorded. The following measurements were taken:
- Mid-sagittal plane: the midline and the widest diameter of the tongue base thickness while stimulation was on compared to the off mode (ΔDsagittal; Figure 4B,C).
- Coronal plane: the tongue base thickness in the midline (ΔDcoronal; Figure 5B,C) as well as the CSA of the tongue, comparing on with off mode (ΔA) (Figure 5D,E).
- Differences in diameters (Δ) were reported in centimeters (cm) and percentual change (%) from baseline, and in square centimeters (cm2) for the CSA.
Statistical analysis
The analysis of the data was carried out using the IBM SPSS 20.0 statistical package software (SPSS Inc., Chicago, IL, USA). Data were expressed as mean and standard deviation (SD) for quantitative parametric measures in addition to both number and percentage for categorized data. ANOVA test was used for comparison between different planes using a post-hoc analysis with Least Significance Difference (LSD) for multiple comparisons. Correlations between the parameters were analysed reporting the Pearson correlation analysis. A P value of 0.05 or less was considered significant. Bland-Altman plots were used to detect bias and 95% limits of agreement between different ultrasound measurements.
Results
The study screened 26 and enrolled 14 patients with OSA [AHI 19.6 (10.7) × hour-1] (Figure 7). The patients were middle-aged, overweight, with more men included. During ES, first sensation of the current was noted at 4.4 (1.5) mA, while contraction of the muscles was achieved at a level of 10.9 (1.1) mA (Table 1).
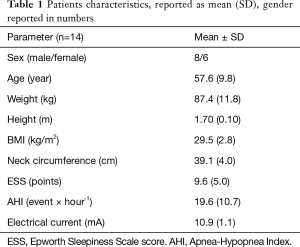
Full table
The mean quality of the ultrasound scans was scored as acceptable or good with 1.5 (0.5) points. The tongue base in the mid-sagittal plane (ΔDsagittal) increased by +12.2% (4.0%) with stimulation (Figure 8; P<0.001). The widest diameter in the sagittal plane increased similarly by +12.9% (5.2%) during stimulation (Figure 9; P<0.001; Table 2).
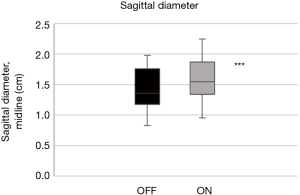
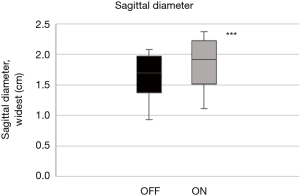
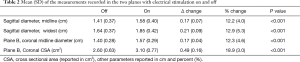
Full table
In the coronal plane, ΔDcoronal increased by +12.3% (4.6%) with stimulation, while ΔAcoronal increased by +18.9% (3.0%) (P<0.001, respectively; Table 2; Figures 10,11).
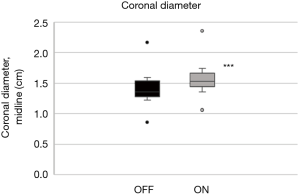
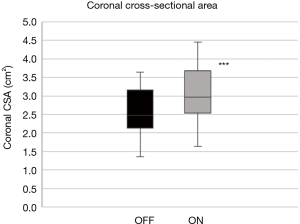
Although similar changes were recorded in the different planes and parameters, there was no significant correlation between them (Table 3).
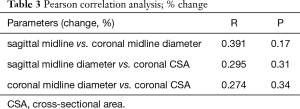
Full table
A Bland-Altman plot was generated to demonstrate the bias between the measurements in two planes (mid-sagittal and coronal diameters). The bias for ΔD in both planes was small [1×10-3 (0.056) cm] and the 95% limits of agreement reached from ‒0.109 to 0.111 cm (Figure 12).
There was a negative correlation between age and ΔA (coronal plane, r= ‒0.6, P=0.03; Table 4).
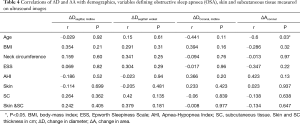
Full table
Discussion
In this study, we found that on analysis of the mid-sagittal and coronal ultrasound images of the submental area in 14 awake seated OSA patients, the tongue base thickness and CSA were significantly increased during the use of the transcutaneous ES. There was small bias for ΔD in both planes (95% limits of agreement: −0.109 to 0.111 cm). Contraction is best detected in the CSA of the tongue base in the coronal plane. Thus, the contraction of the upper airway dilator muscles in direct response to transcutaneous ES can be visualised using ultrasound.
In this context, reproducible measurements of the contraction of the tongue-ground muscles in the mid-sagittal and coronal planes indicate a thickening of the muscles of about 10% (ΔD) (35). Considering the CSA of a muscle with a round model, any contraction leading to an increased diameter of +10% results in an increase of the CSA of about 21% (CSA = π *r2) (35), and this is consistent with the observations of increased dimensions in the coronal plane (ΔAcoronal). The quality of the measurements depends on the individual anatomy, with a large neck circumference making it difficult to position the probe. However, once a good view is achieved, measurements of upper airway dilator muscle contraction can be accurately recorded.
Clinical significance
Previous studies have shown the importance of using different imaging methods (MRI, DISE and ultrasound) in visualisation of the pharynx, the tongue and the upper airway dilator muscles in OSA (38,41,42) evaluating the response of different muscle groups to ES, typically applied via HNS (43), but also non-invasively (30,35,36). In the current study, we demonstrated the use of ultrasound to detect muscle contraction in response to the electrical current delivered transcutaneously in awake patients with OSA. Although ultrasound imaging is non-invasive and allows good visualisation of the pharyngeal structures, standardisation of the technique is challenging. Posture, mouth position (open/closed), wake state, activity (talking/quiet), probe positioning and movement artefacts can make accurate recordings difficult, as indicated by the excluded screened patients. However, we have shown reproducible results indicating the contraction of the upper airway dilator muscles in different planes, indicating that there is little difference in the mid-sagittal and coronal planes. Similarly, the percentual change in the mid-line diameter or the largest diameter in the sagittal plane indicates that both can be chosen for analysis, as long as comparisons are made with the correct reference. Our group has previously used ultrasound scans with similar findings, describing an increase in the distance from skin-to-tongue surface of 10.0% (2.8%) on the sagittal view and 9.4% (3.7%) on the coronal view (35).
Hofauer et al., used sonography to evaluate tongue motions in OSA patients who were using HNS. The tongue was scanned in four planes and, the horizontal and sagittal planes were found to be preferable to the other investigated planes. Tongue motion was evident on the right (implanted) side in 75% in the horizontal plane (44). In our study, we detected motion during muscle contraction in 80%.
Kwan et al., compared ultrasound with magnetic resonance imaging to assess the sagittal measurements of the tongue movement during respiration in twenty-one participants with and without OSA. There was an agreement between the two imaging methods with respect to the anteroposterior tongue motion during inspiration. Ultrasound measurements of the posterior tongue displacement were 0.24±0.64 mm greater than MRI measurements (95% limits of agreement: 1.03 to ‒1.49). The study concluded that ultrasound was a suitable method for measuring tongue movements (45).
In an earlier study, Liu et al measured the lateral para-pharyngeal wall thickness using ultrasound in fifty-eight patients with OSA who had an AHI ≥10× hour–1 and eighteen patients with an AHI <10× hour-1. The sonographic measurements correlated well when compared to magnetic resonance imaging in 15 patients out of the entire cohort (46).
Schwab et al., used computer tomography (CT) imaging during wakefulness in seven patients who responded to HNS and compared it to six non-responders. The patients had baseline scans without HNS, followed by repeat scans with HNS. In the baseline scan it was found that responders to HNS had a smaller soft palate volume and, with stimulation, they had a greater tongue displacement anteriorly, an increased retroglossal airway size, as well as a greater shortening of the mandible-hyoid distance. It was concluded that these findings can be considered as predictors of response to upper airway stimulation. (47). In our study, anterior tongue displacement was reliably observed. Despite difficulty of soft palate volume assessment by ultrasound, further studies are needed to assess the retroglossal airway size and the mandible-hyoid distance (48-50) with observation of changes in response to the ES.
Limitations
This was a small physiological sub-study on the feasibility of a novel approach to assess upper airway muscle dilator response to transcutaneous ES. Although the sample size was small, reproducible measurements were obtained in multiple planes by the investigators. The ultrasound settings, particularly quality of tissue penetration of the picture varied amongst patients due to neck circumference and bulky soft tissue, as well as the anatomical relief allowing the placement of the probe in the right position. A previous lack of standardisation regarding recommendations about positioning and pressure of the applied probe may have further contributed to variability in the measurements. And thus, 12 (46%) out of 26 patients were excluded during the screening process (semi-quantitative score) due to low quality of their ultrasound images. Lastly, the patches used for transcutaneous ES interfered with the placement of the ultrasound probe and this emphasises the importance of an experienced investigator to record reliable measurements when assessing patients. Future studies could compare this method on a larger sample size and test different positions and investigators, as well as standardisation approaches. Furthermore, it would be useful to understand whether the observed effects of ES on the upper airway dilator muscles in the seated posture can be translated to the supine position, as this may be more relevant to the asleep patients. However, none of these confounders negate the relevance of having a ubiquitous tool available at the bedside when screening for responders to ES prior to engaging them for further assessments.
Conclusions
Ultrasound can visualise the upper airway dilator muscles in OSA patients and may serve to identify responders to ES to treat OSA, like HNS and TESLA. It has the advantage of being non-invasive and is widely available at the bedside. Despite limitations when using ultrasound due to qualitative pictures, contraction caused by transcutaneous ES can be determined by measuring the sagittal and coronal diameters of the tongue base. In future studies, it would be useful to combine the non-invasive ultrasound approach with other techniques, such as DISE and MRI, to validate the approach when assessing patients with OSA for novel treatment trials.
Acknowledgments
The views expressed are those of the authors and not necessarily those of the NHS, the NIHR or the Department of Health.
Funding: None.
Footnote
Provenance and Peer Review: This article was commissioned by the Guest Editor (Joerg Steier) for the series “5th Clinical Update Sleep” published in Journal of Thoracic Disease. The article was sent for external peer review organized by the Guest Editor and the editorial office.
Reporting Checklist: The authors have completed the STROBE reporting checklist. Available at http://dx.doi.org/10.21037/jtd-cus-2020-001
Data Sharing Statement: Available at http://dx.doi.org/10.21037/jtd-cus-2020-001
Conflicts of Interest: All authors have completed the ICMJE uniform disclosure form (available at http://dx.doi.org/10.21037/jtd-cus-2020-001). The series “5th Clinical Update Sleep” was commissioned by the editorial office without any funding or sponsorship. JS served as the unpaid Guest Editor of the series and serves as an unpaid editorial board member of Journal of Thoracic Disease. JS’s contribution was partially supported by the National Institute for Health Research (NIHR) Biomedical Research Centre based at Guy’s and St Thomas’ NHS Foundation Trust and King’s College London. The authors have no other conflicts of interest to declare.
Ethical Statement: The authors are accountable for all aspects of the work in ensuring that questions related to the accuracy or integrity of any part of the work are appropriately investigated and resolved. The trial was conducted in accordance with the Declaration of Helsinki (as revised in 2013) and the Harmonised Tripartite Guideline for Good Clinical Practice from the International Conference on Harmonisation. This was a physiological substudy of a randomised controlled trial (NCT03160456) that was approved by the responsible research ethics committee. All patients enrolled completed the informed consent form.
Open Access Statement: This is an Open Access article distributed in accordance with the Creative Commons Attribution-NonCommercial-NoDerivs 4.0 International License (CC BY-NC-ND 4.0), which permits the non-commercial replication and distribution of the article with the strict proviso that no changes or edits are made and the original work is properly cited (including links to both the formal publication through the relevant DOI and the license). See: https://creativecommons.org/licenses/by-nc-nd/4.0/.
References
- Peppard PE, Young T, Barnet JH, et al. Increased prevalence of sleep-disordered breathing in adults. Am J Epidemiol 2013;177:1006-14. [Crossref] [PubMed]
- Steier J, Martin A, Harris J, et al. Predicted relative prevalence estimates for obstructive sleep apnoea and the associated healthcare provision across the UK. Thorax 2014;69:390-2. [Crossref] [PubMed]
- Marin JM, Carrizo SJ, Vicente E, et al. Long-term cardiovascular outcomes in men with obstructive sleep apnoea-hypopnoea with or without treatment with continuous positive airway pressure: An observational study. Lancet 2005;365:1046-53. [Crossref] [PubMed]
- Punjabi NM, Caffo BS, Goodwin JL, et al. Sleep-disordered breathing and mortality: A prospective cohort study. PLoS Med 2009;6:e1000132. [Crossref] [PubMed]
- Benjafield AV, Ayas NT, Eastwood PR, et al. Estimation of the global prevalence and burden of obstructive sleep apnoea: a literature-based analysis. Lancet Respir Med 2019;7:687-98. [Crossref] [PubMed]
- Jordan AS, McSharry DG. Adult obstructive sleep apnoea syndrome. J R Coll Physicians Lond 1999;33:439-44. [PubMed]
- Eckert DJ, Malhotra A. Pathophysiology of adult obstructive sleep apnea. Proc Am Thorac Soc 2008;5:144-53. [Crossref] [PubMed]
- Bosi M, De Vito A, Kotecha B, et al. Phenotyping the pathophysiology of obstructive sleep apnea using polygraphy/polysomnography: a review of the literature. Sleep Breath 2018;22:579-92. [Crossref] [PubMed]
- Romero-Corral A, Caples SM, Lopez-Jimenez F, et al. Interactions between obesity and obstructive sleep apnea: Implications for treatment. Chest 2010;137:711-9. [Crossref] [PubMed]
- Deng X, Gu W, Li Y, et al. Age-group-specific associations between the severity of obstructive sleep apnea and relevant risk factors in male and female patients. PLoS One 2014;9:e107380. [Crossref] [PubMed]
- Simou E, Britton J, Leonardi-Bee J. Alcohol and the risk of sleep apnoea: a systematic review and meta-analysis. Sleep Med 2018;42:38-46. [Crossref] [PubMed]
- Zhu H, Xu H, Chen R, et al. Smoking, obstructive sleep apnea syndrome and their combined effects on metabolic parameters: Evidence from a large cross-sectional study. Sci Rep 2017;7:8851. [Crossref] [PubMed]
- Dempsey JA, Veasey SC, Morgan BJ, et al. Pathophysiology of sleep apnea. Physiol Rev 2010;90:47-112. [Crossref] [PubMed]
- Steier J, Jolley CJ, Seymour J, et al. Increased load on the respiratory muscles in obstructive sleep apnea. Respir Physiol Neurobiol 2010;171:54-60. [Crossref] [PubMed]
- Quinnell TG, Bennett M, Jordan J, et al. A crossover randomised controlled trial of oral mandibular advancement devices for obstructive sleep apnoea-hypopnoea (TOMADO). Thorax 2014;69:938-45. [Crossref] [PubMed]
- de Vries GE, Hoekema A, Doff MHJ, et al. Usage of positional therapy in adults with obstructive sleep apnea. J Clin Sleep Med 2015;11:131-7. [Crossref] [PubMed]
- Spicuzza L, Caruso D, Maria G. Obstructive sleep apnoea syndrome and its management. Ther Adv Chronic Dis 2015;6:273-85. [Crossref] [PubMed]
- Mitchell LJ, Davidson ZE, Bonham M, et al. Weight loss from lifestyle interventions and severity of sleep apnoea: A systematic review and meta-analysis. Sleep Med 2014;15:1173-83. [Crossref] [PubMed]
- Sarkhosh K, Switzer NJ, El-Hadi M, et al. The impact of bariatric surgery on obstructive sleep apnea: A systematic review. Obes Surg 2013;23:414-23. [Crossref] [PubMed]
- MacKay SG, Chan L. Surgical Approaches to Obstructive Sleep Apnea. Sleep Med Clin 2016;11:331-41. [Crossref] [PubMed]
- Sethukumar P, Kotecha B. Tailoring surgical interventions to treat obstructive sleep apnoea: one size does not fit all. Breathe 2018;14:e84-93. [Crossref] [PubMed]
- Sullivan CE, Berthon-Jones M, Issa FG, et al. Reversal of obstructive sleep apnoea by continuous positive airway pressure applied through the nares. Lancet 1981;1:862-5. [Crossref] [PubMed]
- Cao MT, Sternbach JM, Guilleminault C. Continuous positive airway pressure therapy in obstuctive sleep apnea: benefits and alternatives. Expert Rev Respir Med 2017;11:259-72. [Crossref] [PubMed]
- Pengo MF, Czaban M, Berry MP, et al. The effect of positive and negative message framing on short term continuous positive airway pressure compliance in patients with obstructive sleep apnea. J Thorac Dis 2018;10:S160-9. [Crossref] [PubMed]
- Richard W, Venker J, den Herder C, et al. Acceptance and long-term compliance of nCPAP in obstructive sleep apnea. Eur Arch Otorhinolaryngol 2007;264:1081-6. [Crossref] [PubMed]
- Mezzanotte WS, Tangel DJ, White DP. Waking Genioglossal Electromyogram in Sleep Apnea Patients versus Normal Controls (a Neuromuscular Compensatory Mechanism). J Clin Invest 1992;89:1571-9. [Crossref] [PubMed]
- Mezzanotte WS, Tangel DJ, White DP. Influence of sleep onset on upper-airway muscle activity in apnea patients versus normal controls. Am J Respir Crit Care Med 1996;153:1880-7. [Crossref] [PubMed]
- Pengo MF, Steier J. Emerging technology: electrical stimulation in obstructive sleep apnoea. J Thorac Dis 2015;7:1286-97. [PubMed]
- Strollo PJ, Soose RJ, Maurer JT, et al. Upper-Airway Stimulation for Obstructive Sleep Apnea. N Engl J Med 2014;370:139-49. [Crossref] [PubMed]
- Pengo MF, Xiao S, Ratneswaran C, et al. Randomised sham-controlled trial of transcutaneous electrical stimulation in obstructive sleep apnoea. Thorax 2016;71:923-31. [Crossref] [PubMed]
- Bisogni V, Pengo MF, De Vito A, et al. Electrical stimulation for the treatment of obstructive sleep apnoea: a review of the evidence. Expert Rev Respir Med 2017;11:711-20. [Crossref] [PubMed]
- NICE. Hypoglossal nerve stimulation for moderate to severe obstructive sleep apnoea. 2017. Available online: www.nice.org.uk/guidance/ipg598
- Woodson BT, Strohl KP, Soose RJ, et al. Upper Airway Stimulation for Obstructive Sleep Apnea: 5-Year Outcomes. Otolaryngol Head Neck Surg 2018;159:194-202. [Crossref] [PubMed]
- Eastwood PR, Barnes M, MacKay SG, et al. Bilateral hypoglossal nerve stimulation for treatment of adult obstructive sleep apnoea. Eur Respir J 2020;55:1901320. [Crossref] [PubMed]
- Steier J, Seymour J, Rafferty GF, et al. Continuous transcutaneous submental electrical stimulation in obstructive sleep apnea: A feasibility study. Chest 2011;140:998-1007. [Crossref] [PubMed]
- He B, Al-Sherif M, Nido M, et al. Domiciliary use of transcutaneous electrical stimulation for patients with obstructive sleep apnoea: A conceptual framework for the TESLA home programme. J Thorac Dis 2019;11:2153-64. [Crossref] [PubMed]
- Ratneswaran D, Guni A, Pengo MF, et al. Electrical stimulation as therapeutic approach in obstructive sleep apnea—a meta-analysis. Sleep Breath 2020. [Crossref] [PubMed]
- Feng Y, Keenan BT, Wang S, et al. Dynamic upper airway imaging during wakefulness in obese subjects with and without sleep apnea. Am J Respir Crit Care Med 2018;198:1435-43. [Crossref] [PubMed]
- DE Corso E, Fiorita A, Rizzotto G, et al. The role of drug-induced sleep endoscopy in the diagnosis and management of obstructive sleep apnoea syndrome: our personal experience. Acta Otorhinolaryngol Ital 2013;33:405-13. [PubMed]
- Isaiah A, Mezrich R, Wolf J. Ultrasonographic Detection of Airway Obstruction in a Model of Obstructive Sleep Apnea. Ultrasound Int Open 2017;3:E34-42. [Crossref] [PubMed]
- Wang SH, Keenan BT, Wiemken A, et al. Effect of Weight Loss on Upper Airway Anatomy and the Apnea-Hypopnea Index. The Importance of Tongue Fat. Am J Respir Crit Care Med 2020;201:718-27. [Crossref] [PubMed]
- Oliven R, Cohen G, Dotan Y, et al. Alteration in upper airway dilator muscle coactivation during sleep: Comparison of patients with obstructive sleep apnea and healthy subjects. J Appl Physiol 2018;124:421-9. [Crossref] [PubMed]
- Baptista PM, Costantino A, Moffa A, et al. Hypoglossal Nerve Stimulation in the Treatment of Obstructive Sleep Apnea: Patient Selection and New Perspectives. Nat Sci Sleep 2020;12:151-9. [Crossref] [PubMed]
- Hofauer B, Strohl K, Knopf A, et al. Sonographic evaluation of tongue motions during upper airway stimulation for obstructive sleep apnea — a pilot study. Sleep Breath 2017;21:101-7. [Crossref] [PubMed]
- Kwan BCH, Jugé L, Gandevia SC, et al. Sagittal Measurement of Tongue Movement During Respiration: Comparison Between Ultrasonography and Magnetic Resonance Imaging. Ultrasound Med Biol 2019;45:921-34. [Crossref] [PubMed]
- Liu KH, Chu WCW, To KW, et al. Sonographic measurement of lateral parapharyngeal wall thickness in patients with obstructive sleep apnea. Sleep 2007;30:1503-8. [Crossref] [PubMed]
- Schwab RJ, Wang SH, Verbraecken J, et al. Anatomic predictors of response and mechanism of action of upper airway stimulation therapy in patients with obstructive sleep apnea. Sleep 2018;41:1-12. [Crossref] [PubMed]
- Singh M, Chin K, Chan V, et al. Use of Sonography for Airway Assessment An Observational Study. J Ultrasound Med 2010;29:79-85. [Crossref] [PubMed]
- Shu CC, Lee P, Lin JW, et al. The Use of Sub-Mental Ultrasonography for Identifying Patients with Severe The Use of Sub-Mental Ultrasonography for Identifying Patients with Severe Obstructive Sleep Apnea. PLoS One 2013;8:e62848. [Crossref] [PubMed]
- Petrișor C, Trancă S, Szabo R, et al. Clinical versus Ultrasound Measurements of Hyomental Distance Ratio for the Prediction of Difficult Airway in Patients with and without Morbid Obesity. Diagnostics Diagnostics (Basel) 2020;10:140. [Crossref] [PubMed]