Functional residual capacity in beagle dogs with and without acute respiratory distress syndrome
Introduction
Mechanical ventilation was an important supportive strategy for patients receiving surgery or suffering from respiratory failure. Traditionally, the choice of tidal volume, the most important parameter of ventilator settings, was based on the body weight (BW), which was a certain milliliter for per kilogram of BW. Usually, predicted BW was used to correct actual BW inter-individual variations in obesity and muscle weight (1). For patients with acute respiratory distress syndrome (ARDS), low tidal volume (6-8 mL/kg of predicted BW) has become a routine strategy (2,3). This setting has dramatically improved the prognosis (2,4-6). Beyond meeting the requirement of blood gas exchange, the method of selecting tidal volume, according to BW or predicted BW, mainly depended on the fact that normal lung volumes, especially functional residual capacity (FRC), were determined by height (indirectly by predicted BW), sex and age of healthy persons (7). However, FRCs of patients with ARDS might not abide by the same rule. In fact, FRCs might be significantly different from each other in ARDS patients with the same height (in other words, same predicted BW) (8).
It was obvious the rationale of setting tidal volume for normal lungs might be improperly used in injured lungs. Meanwhile, previous studies have demonstrated that ventilator-induced lung injury was attributed to the ratio between tidal volume and FRC but not the ratio between tidal volume and predicted BW (8-10). Therefore, clarifying the relationship between FRC and predicted BW was of significance, especially in injured lungs, which might be helpful to optimize the setting of tidal volume. We hypothesized that FRC was determined by body length (surrogate for predicted BW) and age in healthy beagle dogs but not in lung injured ones.
Methods
Experimental protocol
The research was approved by the Ethics Committee of the First Affiliated Hospital of Zhengzhou University. Twenty-four male beagle dogs weighed 10-14 kg and aged 8.5-14 months were studied in the supine position. FRCs were measured before and after lung injury and then the relationship between FRCs and associated co-variables (age, body length and actual BW) were analyzed. As shown in Figure 1, after surgical preparation and baseline data collections, chest computed tomography (CT) scanning was performed. All of the measurements were performed again after induction of lung injury. If necessary, the ventilation was supported by Evita 4 ventilator (Dräger, Lübeck, Germany) in volume control mode with tidal volume 10 mL/kg of actual BW for healthy animals and 8 mL/kg for ARDS, positive end-expiratory pressure was 5 cmH2O. The other settings were as follows: fraction of inspired oxygen (FiO2), 50%; inspiratory-to-expiratory ratio, 1:2; respiratory rate, 20-40 breaths per minute according to the arterial carbon dioxide tension. During the rest of the time, continuous positive airway pressure was maintained at 5 cmH2O.

Anesthesia and surgical preparation
Preparations were performed according to the method previous described (11). The animals were fasted with free access to water 12 h before the experiment. Anesthesia induction was performed with an intramuscular injection of 10 mg midazolam, 100 mg ketamine hydrochloride and 0.25 mg atropine. A cuffed endotracheal tube (7.5 mm inner diameter) was inserted in the airway. The right femoral vein was cannulated with a single lumen catheter (Arrow International, Inc., Reading, PA) for anesthesia, fluid, and drug infusion. General anesthesia was maintained with 5-10 mg/kg/h propofol and 0.25-0.5 mg/kg/h cisatracurium besylate through a syringe pump (SN-50C6; Sinomdt, Shenzhen, China). The left femoral artery was cannulated with a 4F PiCCO Pulsiocath catheter to monitor the arterial blood pressure and core temperature, calculate extra vascular lung water index by PiCCO plus (Pulsion Medical Systems, Munich, Germany), and obtain arterial blood samples for gas analysis. Vital signs such as pulse oxygen saturation of tongue, respiratory rate, heart rate, and electrocardiograph were monitored by a monitor (PM-9000Vet, Mindray, Shenzhen, China).
Measuring FRC
Before the measurement, animals were ventilated in volume controlled mode. Dogs were disconnected from ventilation support without spontaneous breath under full sedation and muscle relaxant for a second (airway pressure 0 cmH2O). CT scanning was performed quickly before and after lung injury with Siemens Definition 128 slices, and the acquisition software syngo CT 2008G (Siemens HealthCare, Erlangen, Germany) was used (11). Images were analyzed with the dedicated software (LungCAD, Neusoft, Shenyang, China). The density of lung parenchyma was assumed to be close to the density of water (0 Hounsfield Units, HU) and each voxel to be composed of lung tissue (0 HU) and air (−1,000 HU). Gas fraction in each voxel was computed as: mean CT number/(CT number gas—CT number tissue) = voxel density/−1,000. Gas volume in one voxel was the voxel volume multiplied by gas fraction. FRC was computed as the sum of gas volumes of all voxels in the whole lung. Aeration of lung parenchyma was classified as: lung density >−100 HU (not inflated tissue), −100 to −500 HU (poorly inflated tissue), −500 to −900 HU (well inflated tissue) and <−900 HU (over inflated tissue). The parameters of CT scans were as follows: slice thickness, 2 mm; X-ray tube current, 110 mA; collimation width, 64 mm × 2 mm × 0.6 mm; reconstruction interval, 3 mm; data collection field of view, 500 mm; kVp, 100; pixel dimensions, 0.58/0.58; reconstruction field of view, 180 mm and acquisition matrix 512×512. All measurements were performed in the same conditions, which wouldn’t influence the observed value.
Introduction of lung injury
Under the ventilation support with continuous positive airway pressure mode (5 cmH2O), 99% oleic acid (Sigma-Aldrich, California, USA) was diluted in 20 mL of ethanol to make model (11,12) and arterial blood gas was analyzed with ABL800 (Radiometer, Copenhagen, Denmark) until the ratio of the partial pressure of oxygen in arterial blood to FiO2 (PaO2/FiO2) was lower than 200 mmHg and did not improve within 30 min to meet the PaO2/FiO2 diagnostic criteria of moderate ARDS with CPAP at 5 cmH2O (13). If necessary, dopamine was given at 5-10 μg/kg/min to guarantee mean arterial pressure >60 mmHg.
Statistical analysis
Data were presented as means ± standard error. Gas exchange, respiratory mechanics, hemodynamic parameters and imaging presentation before and after lung injury were compared using paired samples t-test. Multiple linear regressions were performed to analyze the relationship between FRC and age, length and actual BW. All tests were performed using SPSS 16.0 (SPSS Inc., Chicago, IL, USA). A P value <0.05 was regarded as statistically significant. The graph was plotted with dedicated software, Origin 8.0 (Originlab, Massachusetts, USA).
Results
Blood gas analysis
As shown in Table 1, the ratio of PaO2/FiO2 decreased sharply from 472.4±58.9 mmHg at baseline to 166.2±19.1 mmHg after venous injection of oleic acid (P<0.001). Arterial blood pHa decreased and carbon dioxide tension increased significantly with HCO3− being stable.
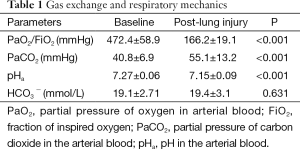
Full table
Hemodynamics
Extravascular lung water index increased from (11±1.9) to (25.7±7.9) mL/kg (P<0.001). Cardiac index and heart rate decreased significantly after the induction of lung injury (P<0.001). There were no dramatically change in mean arterial pressure and central venous pressure (P>0.05, Table 2).
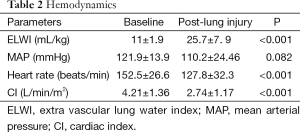
Full table
Imaging presentation
Table 3 showed the typical imaging characteristics of ARDS. The lung gas volume, percentage of well-inflated and overinflated lung tissue decreased significantly, while lung density, lung weight, percentage of not inflated and poorly inflated tissues increased dramatically, and the total lung volume did not alter notably.
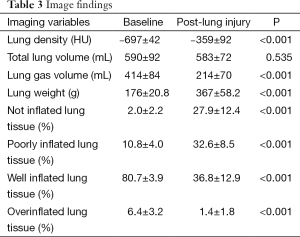
Full table
The relationship between FRC and length, age and actual BW of dogs without ARDS
As shown in the 3D scatter graph (Figure 2), FRCs in dogs without ARDS increased with age (11.5±1.7 months) and body length (73±1.876 cm). When the independent variables such as age, length and actual BW (12.2±1.2 kg) were examined by multiple linear regression technique, the formula, FRC =21.86× age (months) +20.55× length (cm) –1,337.98 (P<0.005), provided a good estimate of the FRC in healthy supine individuals. However, actual BW was not considered as an independent variable (P=0.993), and the relationship between FRC and actual BW was not proportional (Figure 3).
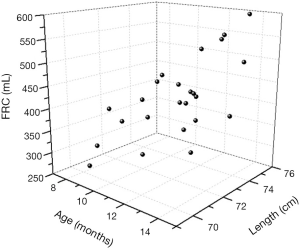
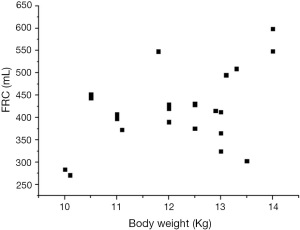
The relationship between FRC and age, length and actual BW of dogs with ARDS
As shown in the 3D scatter graph (Figure 4), FRCs in dogs with ARDS did not change with age and body length as in healthy status. There was no linear relationship between FRC and any 1 of the 3 independent variables, and the formula of multiple linear regression was invalid (P=0.305). The relationship between FRC and length was unpredictable, and the difference of FRC between individuals was also tremendous for the actual BW (Figure 5).
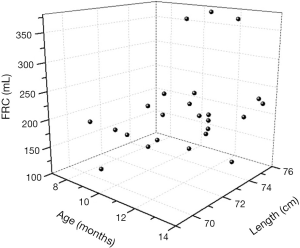
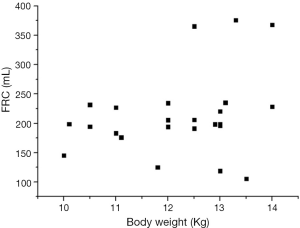
Discussion
In this study, we have made a typical animal model of pulmonary ARDS, which has simulated the major characteristics of moderate ARDS. We demonstrated for the first time that there was a multivariate linear relationship between FRC and body length (surrogate for predicted BW) and age in healthy dogs, but not in dogs with ARDS.
The present ARDS animal model bore the following characteristics: (I) acute onset with a known clinical insult (oleic acid), which took 190±58 min to make the lung injury model; (II) hypoxia, the ratio of PaO2/FiO2 was between 100 and 200 mmHg with positive end-expiratory pressure 5 cmH2O, and the mean was 166.2 mmHg; (III) typical imaging presentation of ARDS (14) as shown in Figure 6; (IV) alveolar edema, extra vascular lung water index increased from the baseline (11±1.9) to (25.7±7.9) mL/kg. This model had mimicked the typical and basic features of human ARDS in disease onset, oxygenation (13), imaging (14), and hemodynamics (15). Pilot experiments were performed and sample size was estimated by means and standard error of FRC and the number of variable in the multiple regression analysis (16,17). All of these laid a solid foundation for our study.
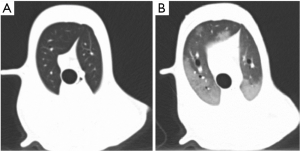
In our study, FRC was measured with CT scan, a golden standard method (7,18). The detection error wouldn’t bias the result significantly. In order to reduce the effect of tidal volume on recruitment, which might increase the differences of FRC between both experimental conditions, the ventilation was supported with different tidal volume, smaller for the injured lungs in consideration of the relative smaller lung volumes. In addition, we introduced body length to surrogate for predicted BW. Until now, there was no recognized formula to describe the relationship between predicted BW and body length in beagle dogs as in human beings (7). However, previous study informed us that BW of beagle dog increased with body length until being adult (19). It is sure enough that the larger the body length, the heavier the predicted BW, which can be delegated by body length in a certain extent. As shown in the present study, FRC is mainly affected by age and body length for the healthy dogs. Additionally, the formula was valid when analyzed with multiple linear regressions, but actual BW was not considered as a valid independent variable. Figure 2 has shown that FRC would increase with the increase of age and length. In other words, FRC was proportional to age and body length to some extent. However, the law did not exist between FRC and actual BW (Figure 3), which was consistent with our previous findings (11). Moreover, the study in human normal lungs demonstrated that FRC varied from about 20-46 mL/kg of predicted BW at 5 cmH2O of positive end-expiratory pressure. Therefore, supposing a healthy person with 60 kg predicted BW, the FRC may vary from 1,200-2,760 mL (20).
After lung injury, FRC dramatically decreased as in human studies (20,21), although PaO2/FiO2 of each model was within the boundaries of moderate ARDS, the inter-individual variation of FRC was significant. We did not find any rules to follow in the relationship between FRC and age or length, and FRC was not relevant to actual BW either. Until now, there was no any research with targeted objective to explore the relationship between FRC and predicted BW or equivalent (such as height). Chiumello et al. (21) recruited 24 ARDS patients with height 172±9 cm, the standard deviation of height was small, only 5.2% of the mean. However, the standard deviation of FRC (1,013±593 mL) was large, 58.5% of the mean. The study informed us that the ratio of FRC to height or predicted BW was not rigidly fixed but fickle. Another study with 40 patients with ARDS showed a mean value of FRCs of 16 mL/kg of predicted BW, with minimal and maximum values being 13 mL/kg of predicted BW and 22 mL/kg of predicted BW, respectively (21).
In traditional mechanical ventilation strategy, tidal volume was adjusted between 10 and 15 mL/kg of BW to achieve normal values for the pH, gas exchange (22). Low tidal volume (6-8 mL/kg of BW) tailored for “protective ventilation” was accepted generally (2,4-6) considering lung volumes reduced by atelectasis and lung edema in patients suffering from ARDS. Usually, to correct actual BW “inter-individual variations” in obesity and muscle weight, predicted BW was introduced. A critical question was why we should select tidal volume based on BW or predicted BW regardless of healthy lungs or injured ones. The principle might rely on the fact that normal lung volumes, especially FRC, are dependent on sex and proportional to height (indirectly proportional to predicted BW) in healthy individuals (7). It should not be ignored that law is just true in healthy lungs. The tidal volume tailored for ARDS patient has decreased about 40% from the one tailored for “healthy” person. The rationality would exist if FRC also decreased about 40% after acute lung injury. However, as shown in our study, there is no proportional relationship between FRC and length or actual BW despite the model possessing a high degree of similarity in many aspects. The further analysis showed that FRCs of this ARDS model was relevant to PaO2/FiO2 (Figure 7). In clinical settings, the severity of ARDS, the underlying diseases and comorbidities were different from each other. Therefore, lung volumes are impossible to reduce in a constant proportion. For an 80 kg patient, the FRC may vary from 200-800 mL (8). In the choice of tidal volume, it is possible that we have improper use of the rule-governed in healthy lung on the disease status. Volutrauma and barotrauma are primarily due to unphysiological lung strain (the ratio between tidal volume and FRC) but not the ratio between tidal volume and predicted BW or height (9). All of these implied that more attention should be paid to the actual lung volume (especially FRC) or the ratio of tidal volume to FRC.
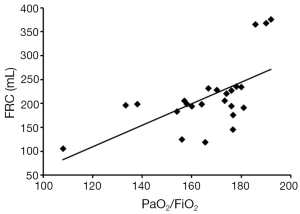
There are some limitations in our study. Firstly, ARDS model was induced with oleic acid, which was different from the most common pathogenic factor (infectious agents) in clinical settings, result the different physiopathologic changes. Secondly, animals were studied in the “supine” position, but dogs do not stand upright as human, the long axis of lungs is paralleled to the floor while it is vertical in human. Thus, the effect of superimposed gravity is different, which may affect the result slightly. Thirdly, FRC is the lung volume at the end of expiration during spontaneous breathing. Limited by the cooperation for measuring FRC, sedatives and muscle relaxant were adopted, and spontaneous breathing was interrupted during the measurements that may distorted the actual relationship.
In conclusion, FRC was linearly related to body length (surrogate for predicted BW) in healthy dogs but not in lung injured ones. The method of selecting tidal volume based on predicted BW should be challenged cautiously. More attention should be paid to the actual lung volume (especially FRC) or the ratio of tidal volume to FRC but not only the ratio of tidal volume to predicted BW.
Acknowledgements
We are grateful to Qingsi Zeng and Huai Chen (from Department of Radiology, the First Affiliated Hospital of Guangzhou Medical University) for valuable technical support. We thank Thomas Bice (from Division of Pulmonary and Critical Care medicine, School of Medicine, the University of North Carolina) for review this paper.
Funding: This work was supported by National Nature Science Foundation of China [Project Number: 81400051].
Footnote
Conflicts of Interest: The authors have no conflicts of interest to declare.
References
- Günther A, Taut F. Tidal volume in mechanical ventilation: the importance of considering predicted body weight. Am J Respir Crit Care Med 2008;178:315-6. [PubMed]
- Ventilation with lower tidal volumes as compared with traditional tidal volumes for acute lung injury and the acute respiratory distress syndrome. The Acute Respiratory Distress Syndrome Network. N Engl J Med 2000;342:1301-8. [PubMed]
- Jaswal DS, Leung JM, Sun J, et al. Tidal volume and plateau pressure use for acute lung injury from 2000 to present: a systematic literature review. Crit Care Med 2014;42:2278-89. [PubMed]
- Chen YF, Lim CK, Ruan SY, et al. Factors associated with adherence to low-tidal volume strategy for acute lung injury and acute respiratory distress syndrome and their impacts on outcomes: an observational study and propensity analysis. Minerva Anestesiol 2014;80:1158-68. [PubMed]
- Petrucci N, De Feo C. Lung protective ventilation strategy for the acute respiratory distress syndrome. Cochrane Database Syst Rev 2013;2:CD003844. [PubMed]
- Putensen C, Theuerkauf N, Zinserling J, et al. Meta-analysis: ventilation strategies and outcomes of the acute respiratory distress syndrome and acute lung injury. Ann Intern Med 2009;151:566-76. [PubMed]
- Gommers D. Functional residual capacity and absolute lung volume. Curr Opin Crit Care 2014;20:347-51. [PubMed]
- Gattinoni L, Pesenti A. The concept of "baby lung". Intensive Care Med 2005;31:776-84. [PubMed]
- Protti A, Cressoni M, Santini A, et al. Lung stress and strain during mechanical ventilation: any safe threshold? Am J Respir Crit Care Med 2011;183:1354-62. [PubMed]
- Gattinoni L, Carlesso E, Caironi P. Stress and strain within the lung. Curr Opin Crit Care 2012;18:42-7. [PubMed]
- Liu Q, Li W, Zeng QS, et al. Lung stress and strain during mechanical ventilation in animals with and without pulmonary acute respiratory distress syndrome. J Surg Res 2013;181:300-7. [PubMed]
- Matute-Bello G, Frevert CW, Martin TR. Animal models of acute lung injury. Am J Physiol Lung Cell Mol Physiol 2008;295:L379-99. [PubMed]
- ARDS Definition Task Force. Acute respiratory distress syndrome: the Berlin Definition. JAMA 2012;307:2526-33. [PubMed]
- Gattinoni L, Caironi P, Valenza F, et al. The role of CT-scan studies for the diagnosis and therapy of acute respiratory distress syndrome. Clin Chest Med 2006;27:559-70. abstract vii. [PubMed]
- Kushimoto S, Taira Y, Kitazawa Y, et al. The clinical usefulness of extravascular lung water and pulmonary vascular permeability index to diagnose and characterize pulmonary edema: a prospective multicenter study on the quantitative differential diagnostic definition for acute lung injury/acute respiratory distress syndrome. Crit Care 2012;16:R232. [PubMed]
- Helland IS. On the Interpretation and Use of R2 in Regression Analysis. Biometrics 1987;43:61-9.
- Bonett DG, Wright TA. Sample size requirements for multiple regression interval estimation. J Organiz Behav 2011;32:822-30.
- Heinze H, Eichler W. Measurements of functional residual capacity during intensive care treatment: the technical aspects and its possible clinical applications. Acta Anaesthesiol Scand 2009;53:1121-30. [PubMed]
- Wang X, Chen Y, Xie LL, et al. The characteristics of biology and electrocardiogram in normal beagles. Chinese Journal of Comparative Medicine 2007;17:136-8.
- Dellamonica J, Lerolle N, Sargentini C, et al. Effect of different seated positions on lung volume and oxygenation in acute respiratory distress syndrome. Intensive Care Med 2013;39:1121-7. [PubMed]
- Chiumello D, Carlesso E, Cadringher P, et al. Lung stress and strain during mechanical ventilation for acute respiratory distress syndrome. Am J Respir Crit Care Med 2008;178:346-55. [PubMed]
- Marini JJ. Evolving concepts in the ventilatory management of acute respiratory distress syndrome. Clin Chest Med 1996;17:555-75. [PubMed]