Risk factors, complications and costs of prolonged air leak after video-assisted thoracoscopic surgery for primary lung cancer
Introduction
Prolonged air leak (PAL) is a common complication after lung resection. Reported PAL rates vary from 3.5% to 25.5%, with a higher rate when lobectomy was performed rather than a segmentectomy or wedge resection (1-5). After Lung Volume Reduction Surgery (LVRS), even up to every second patient suffers from PAL (6). Overextension of the residual lung tissue after lung resection might be the main reason for PAL, with LVRS patients representing a patient group with highly emphysematous lung tissue (1). Moreover, PAL patients show a higher rate of pneumonia and empyema, adding time to the postoperative recovery process (7). As a subsequent result, patients with PAL have a significantly longer length of stay (LOS). It has, therefore, also an impact on socio-economic aspects (8).
Even after successful discharge, a recurrent pneumothorax is the second most common reason for readmission to the hospital (9). The associated complications, extended LOS and elevated readmission rates lead to a more significant financial burden on modern health care systems through an accumulation of hospital days and extended treatment regimes.
This study aims to explore, exhibit and discuss risk factors, complications and excessive cost of PAL by analyzing our institutional database on video-assisted thoracoscopic surgery (VATS) patients. We present the following article in accordance with the STROBE reporting checklist (available at https://jtd.amegroups.com/article/view/10.21037/jtd-21-2011/rc).
Methods
All patients scheduled for anatomical VATS resection for lung cancer at our department were studied for data analysis. The database is prospectively maintained and includes all patients from the introduction of our VATS program in February 2009 until March 2021. The study was conducted in accordance with the Declaration of Helsinki (as revised in 2013). The study was approved by the ethics committee of the Medical University of Innsbruck (registration number: AN5163 327/4.17 382/5.2) and individual consent for this retrospective analysis was waived.
A total of 979 patients were screened. Pneumonectomy was defined as the sole exclusion criteria, resulting in 957 patients remaining for further analysis, as is depicted in Figure 1. Recorded data included but was not limited to age, gender, type of operation, complications, length of operation, chest drain duration, LOS, follow-up in the outpatient department. PAL was defined as an air leak lasting for 5 days or longer. Air leak was diagnosed either with “Thopaz+ Digital Chest Drainage and Monitoring System” (Medela AG, Baar, Switzerland) and a flow of >20 mL/minute or through visual detection of air bubbles in conventional water seal chamber systems.
For 311 patients, radiological data measuring the skeletal muscle index was available and was used for analyzing skeletal muscle status. Skeletal muscle area was measured at the third lumbar vertebra. Sarcopenia was defined according to Derstine et al. as a skeletal muscle index <34.4 cm2/m2 for women and <45.4 cm2/m2 for men (10).
Surgical management
VATS surgery was performed with a standardized three-port approach using the Copenhagen technique (11). All surgeries were performed with the use of mechanical staplers for closing bronchi and stapling lung parenchyma. All patients received at least one chest tube at the end of the procedure (20–24 French) connected to a conventional water seal chamber system. Chest tubes were not removed before at least 1 day after surgery. Criteria for chest tube removal at our department are no visual detection of air bubbles in the water seal chamber or measured air flow ≤20 mL/minute and <300 mL of drainage amount over the course of 24 hours.
In case of air leak the conventional water seal chamber system was changed for the Thopaz+ system with a suction rate of 10 cmH2O.
Indication for re-operation was decided individually for each patient based on the clinical course and size of PAL. If a minor parenchymal PAL was suspected (moderate air flow on digital chest drain), decision was usually postponed until postoperative day 12–14, as many of these air leaks resolve spontaneously. If the course of the patient was suspicious for a bronchopleural fistula (high air flow on digital chest drain), reoperation was usually performed earlier. Re-operation was primarily performed by VATS and intraoperative surgical strategies included wedge resection of the parenchymal defect, fibrin glue, talcum pleurodesis, pleural sutures and completion lobectomies. According to our inhouse standards, no patient was discharged with any kind of temporary drainage system in place.
Statistical analysis
Patients were grouped in a PAL and a non-PAL cohort. For cost estimation data of four publications was used. These publications were found with a search syntax, which was used for querying MEDLINE, PubMed Central and Bookshelf: “(prolonged [tiab]) AND (air [tiab]) AND (leak [tiab] OR fistula [tiab]) AND (cost [tiab] OR readmission [tiab]) AND (lung [tiab] OR pulmonary [tiab] OR lobe [tiab] OR segment* [tiab] OR pneumothor* [tiab] OR lobar [tiab] OR lobectom* [tiab] OR segmentectom* [tiab]) AND (surgery [tiab] OR resection [tiab])”. Cost estimation was based on LOS only. Statistical analysis was performed using IBM SPSS Statistics 26 (IBM Corporation, Armonk, NY, USA). Pearson’s chi-squared and Fisher’s exact test were used for identifying correlations between categorical variables. T-test was performed to compare mean values between groups; Kolmogorov-Smirnov test was used for analyzing distribution; Mann-Whitney U test was used to compare medians. For binomial logistic regression location of resection was divided in a dichotomous variable, depending on if the location of the resection included the right upper lobe. According to literature right upper lobe location resection represents a major risk factor for PAL, as one of the pathophysiological hypotheses for development of PAL is an overdistension of remaining lung tissue (1,5,12-14). Binomial logistic regression was used for calculating a risk prediction model for the development of PAL. Statistical significance was assumed for a P value <0.05.
Results
Our cohort consisted of 957 patients, scheduled for a primary anatomical VATS resection, with 103 suffering from PAL (10.8%). The PAL cohort had a significantly higher rate of male patients than the non-PAL cohort (73.8% vs. 49.6%, P<0.001) with a lower body mass index (BMI) of 24.0 vs. 25.5 kg/m2 (P<0.001), and patients suffering from PAL were of higher age (66.2 vs. 63.5 years, P=0.022). Patients’ demographics are shown in Table 1.
Table 1
Factor | Total (n=957) | PAL (n=103) | Non-PAL (n=854) | P value | |
---|---|---|---|---|---|
Mean age (years) (min–max) | 63.8 (13.0–85.5) | 66.2 (31.6–82.3) | 63.5 (13.0–85.5) | 0.022 | |
Sex, n (%) | <0.001 | ||||
Female | 457 (47.8) | 27 (26.2) | 430 (50.4) | ||
Male | 500 (52.2) | 76 (73.8) | 424 (49.6) | ||
Mean body weight (kg) (min–max) | 73.6 (37.5–147.0) | 71.7 (47.0–114.0) | 73.8 (37.5–147.0) | 0.138 | |
Mean size (cm) (min–max) | 170.1 (145.0–196.0) | 172.6 (147.0–190.0) | 169.9 (145.0–196.0) | 0.003 | |
Mean BMI (kg/m2) (min–max) | 25.3 (13.3–48.6) | 24.0 (16.4–35.6) | 25.5 (13.3–48.6) | <0.001 | |
Sarcopenia, n (%)* | 78 (25.1) | 20 (48.8) | 58 (21.5) | <0.001 | |
COPD, n (%) | <0.001 | ||||
No COPD | 646 (67.5) | 49 (47.6) | 597 (69.9) | ||
GOLD stage I | 64 (6.7) | 7 (6.8) | 57 (6.7) | ||
GOLD stage II | 222 (23.2) | 44 (42.7) | 178 (20.8) | ||
GOLD stage III | 24 (2.5) | 3 (2.9) | 21 (2.5) | ||
GOLD stage IV | 1 (0.1) | 0 (0) | 1 (0.1) | ||
Location of resection, n (%) | 0.034 | ||||
Left upper lobe | 207 (21.6) | 23 (22.3) | 184 (21.5) | ||
Right upper lobe | 290 (30.3) | 41 (39.8) | 249 (29.2) | ||
Left lower lobe | 154 (16.1) | 13 (12.6) | 141 (16.5) | ||
Right middle lobe | 58 (6.1) | 2 (1.9) | 56 (6.6) | ||
Right lower lobe | 208 (21.7) | 17 (16.5) | 191 (22.4) | ||
Upper bilobectomy | 15 (1.6) | 1 (1.0) | 14 (1.6) | ||
Lower bilobectomy | 25 (2.6) | 6 (5.8) | 19 (2.2) | ||
Location of resection subgroup analysis, n (%) | 0.044 | ||||
Resection involving right upper lobe | 305 (31.9) | 42 (40.8) | 263 (30.8) | ||
No resection involving right upper lobe resection | 652 (68.1) | 61 (59.2) | 591 (69.2) | ||
Mean time of operation (minutes) (min–max) | 163.1 (42.0–494.0) | 179.1 (65.0–323.0) | 161.2 (42.0–494.0) | 0.001 | |
Mean days until removal of chest drains (min–max) | 5.4 (1.0–62.0) | 14.2 (5.0–62.0) | 4.4 (1.0–20.0) | <0.001 | |
Mean length of stay (days) (min–max) | 10.4 (2.0–117.0) | 19.8 (6.0–117.0) | 9.3 (2.0–87.0) | <0.001 | |
Postoperative infections, n (%) | 137 (14.3) | 32 (31.1) | 105 (12.3) | <0.001 | |
Reoperation during stay as a result of an air leak, n (%) | 14 (1.5) | 12 (11.7) | 2 (0.2) | <0.001 |
*, data available for 311 patients only. PAL, prolonged air leak; BMI, body mass index; COPD, chronic obstructive pulmonary disorder; GOLD, Global Initiative for Chronic Obstructive Lung Disease.
Besides the already mentioned risk factors above, PAL was associated with a preoperative diagnosis of chronic obstructive pulmonary disease (COPD) (52.4% vs. 30.1%, P<0.001), with 17.4% of all COPD patients suffering from PAL (vs. 7.6%, P<0.001) and a correlating decreased forced expiratory volume in 1 second in percent of the predicted value (FEV1, 74.4% vs. 82.5%, P<0.001). Distribution of COPD stages can be seen in Figure 2. The PAL cohort had a significantly higher rate of patients with sarcopenia (48.8% vs. 21.5%, P<0.001). The rate of neoadjuvant therapy did not differ between groups (15.5% vs. 16.9%, P=0.889).
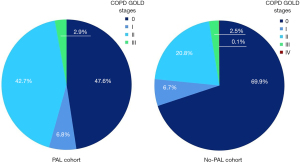
Analyzing the resection location, the right upper lobe resection rate was significantly higher in the PAL cohort (39.8% vs. 29.2%, P=0.034). PAL patients presented with a significantly longer operation time of 179.13 vs. 161.19 minutes (P=0.001).
The PAL cohort showed a significantly longer duration until removal of the last chest drain with a more than threefold increase of 14.21 vs. 4.36 days (P<0.001). Correspondingly, the LOS was elevated from 9.26 days in the non-PAL cohort to 19.84 days in the PAL cohort (P<0.001), as shown in Figure 3.
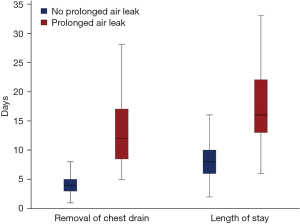
PAL was also associated with a higher risk for postoperative infections with an OR of 3.211 (31.1% vs. 12.3%, P<0.001). Regarding severity of complications, 41.7% of the PAL cohort had a Clavien-Dindo classification of at least three, compared to 8.8% in the non-PAL cohort (P<0.001). Out of 854 patients in the non-PAL group, one patient had a reoperation due to suspected bronchopleural fistula in comparison to seven out of 103 in the PAL group. In case of suspicion for bronchopleural fistula reoperation was performed 3.38 days after primary surgery (vs. 13.00 days for parenchymal fistula, P<0.001). The rate of reoperation due to an air leak was also significantly higher for the PAL cohort with an OR of 56.176 (11.7% vs. 0.2%, P<0.001). Reoperated patients showed to have a significantly prolonged LOS (28.36 vs. 10.13 days, P=0.021). Subgroup analysis of the PAL cohort showed a prolonged LOS in reoperated patients, but failed to reach significance (29.92 vs. 18.51 days, P=0.186). In 13 (92.9%) patients, parenchymal fistula was confirmed and in 1 (7.1%) bronchopleural fistula.
Cost analysis
For calculating the costs of the (excessive) LOS on the normal ward for our cohort, the reported daily costs by Fung et al. [2021], Brunelli et al. [2020], Brunelli et al. [2018] and Varela et al. [2005] were used. Values in US-Dollar were converted for a rate of 1.1442 $ equalling 1 € (15-18).
As the LOS was significantly longer in the PAL cohort, the cost of the calculated LOS was also naturally significantly higher. The mean calculated costs for our cohorts ranged from 2,888.2 to 12,342.8 € for PAL patients and from 1,370.5 to 5,856.8 € for non-PAL patients, as can be seen in Table 2 and Figure 4.
Table 2
Source of daily costs | Total (n=957), mean (min–max) | PAL (n=103), mean (min–max) | Non-PAL (n=854), mean (min–max) | P value |
---|---|---|---|---|
Fung et al. (15)—148 € | 1,542.7 (296.0–17,316.0) | 2,888.2 (888.0–17,316.0) | 1,370.5 (296.0–12,876.0) | <0.001 |
Brunelli et al. (16)—343 € | 3,575.4 (686.0–40,131.0) | 6,693.5 (2,058.0–40,131.0) | 3,176.2 (686.0–29,841.0) | <0.001 |
Brunelli et al. (17)—374.06 € | 3,899.2 (748.1–43,765.0) | 7,299.6 (2,244.4–43,765.0) | 3,463.8 (748.1–32,543.2) | <0.001 |
Varela et al. (18)—632.49 € | 6,593.0 (1,265.0–74,001.3) | 12,342.8 (3,794.9–74,001.3) | 5,856.8 (1,265.0–55,026.6) | <0.001 |
PAL, prolonged air leak.
The mean estimated costs after stratifying for reoperation due to an air leak were significantly higher for the reoperated cohort with 4,196.86 to 17,935.61 € (vs. 1,494.28 to 6,385.94 €, P=0.020). It is important to note, that these costs do not include the additional costs of reoperations.
Risk prediction model
After performing univariate analysis, significant dichotomous and interval-ratio variables (age, sex, size, BMI, COPD and upper lobe resection) have been used to perform binomial logistic regression. Sarcopenia was not used for model creation, as data was only available for 311 patients (32.5%). All variables, except size, showed a significant impact for binomial logistic regression, as can be seen in Table 3.
Table 3
Factor | B | Significance | Exp(B) |
---|---|---|---|
Age | 0.022 | 0.044 | 1.022 |
Sex | 1.016 | 0.001 | 2.763 |
Size | 0.007 | 0.686 | 1.007 |
BMI | −0.110 | 0.000 | 0.896 |
COPD | 0.699 | 0.001 | 2.011 |
Resection involving right upper lobe | 0.501 | 0.025 | 1.651 |
Constant | −3.076 | 0.313 | 0.046 |
BMI, body mass index; COPD, chronic obstructive pulmonary disorder; PAL, prolonged air leak.
Being male increases the odds of suffering from a PAL after anatomical VATS resection by 176%, suffering from COPD by 101%, resection involving right upper lobe by 65%, each year of age by 2% and each cm of body size by 0.7% (not significant). In contrast every unit of BMI decreases the risk of PAL by 10%.
According to these results a risk prediction formula for the development of PAL was established: odds = econstant + 0.022× age +1.016× sex (male =1) + 0.007× size (cm) −0.110× BMI +0.699× COPD (yes =1) +0.501× resection involving right upper lobe (yes=1).
For example, a female patient, 65 years of age, body size of 172 cm, a BMI of 23 kg/m2, with COPD and a tumour requiring a resection involving the right upper lobe the odds for a PAL are: e−3.076+0.022×65+1.016×0+0.007×172−0.110×23+0.699×1+0.501×1=0.1700. Accordingly, the probability [= odds/(1 + odds)] for this patient to develop a PAL would be: 0.1700/(1+0.1700) =0.1453. In other words, there is a 14.53% chance for this patient to develop a PAL after anatomical VATS resection for primary lung cancer.
Discussion
PAL remains one of the most common postoperative complications after lung resections with various treatment options and opinions (1-6). Accordingly, PAL is responsible for postoperative readmissions and elevated treatment costs. Our paper aims to provide an analysis of our department’s data regarding PAL. The rate of PAL in our cohort was 10.8%, which is in line with reported numbers in the available literature ranging from 3.5% to 25.5% (1-5).
Pathophysiology and risk factors of PAL have been studied many times before. The pathophysiology behind PAL after surgical resection is most likely due to residual space in the pleural cavity after resection of lung parenchyma, which is filled by the remaining lung tissue. The possible overdistension of the remaining lung and its alveoli increases the traction on staple lines (1). This also explains the higher rate of air leak in emphysematous lungs, e.g., in patients receiving LVRS, and the higher rate of PAL after lobectomy (1,19).
As a result, upper lobe lobectomy poses a risk factor for PAL because of the associated larger residual space with a meta-analysis by Pan et al. showing an OR of 2.03 for a European and American patient population (P<0.001 in the fixed effect model); the Asian cohort did not present with a higher risk for PAL when upper lobectomy was performed. Abolhoda et al. even report a 25.5% PAL rate in their radical upper lobectomy cohort, and Brunelli et al. show that 74.1% of lobectomy/bilobectomy patients with PAL received an upper resection (5,12-14). Also, in our analysis the rate of upper lobe resections was significantly higher in the PAL cohort (63.1% vs. 52.3%, P=0.047).
Many other risk factors for developing a PAL have been identified, with some being (partly) directly influenceable by the course of the surgery itself. Pleural adhesions show significant association with postsurgical PAL being present in 50.6% of PAL patients (vs. 30.1%; P=0.0002), and Pan et al. calculated an OR of 1.96 (P<0.001) for their overall cohort (12). This seems to be due to the higher needed amount of mobilization and often blunt dissection causing injuries to the visceral pleura. Yotsukura et al. endorse these risk factors with their cohort of 91 PAL and 2,187 non-PAL patients, in which lobectomy/bilobectomy, pleural adhesions, male sex and smoking history of >20 pack years correlate with PAL (OR 1.935, P=0.025; OR 3.899, P<0.001; OR 2.269, P=0.013; OR 2.441, P=0.005) (20). Unfortunately, pleural adhesions were not constantly documented in our cohort.
While Pan et al. showed longer stapler line length being a risk factor for PAL (210.0 vs. 145.5 mm; P<0.001), Brunelli et al. did not find a significant difference between cohorts (12,13).
In our cohort, COPD as a surrogate parameter for the quality of a patient’s lung tissue, was associated with a higher rate of PAL (17.4% vs. 7.6%, P<0.001). Moreover, the PAL cohort had a significantly decreased FEV1% (74.4% vs. 82.5%, P<0.001) and FEV1 to FVC ratio (68.4% vs. 76.5%, P<0.001). Non-surgical risk factors are also linked to the lung parenchyma and its function. According to Liang et al., emphysema shows to be a risk factor, with 49.2% of PAL patients having signs of emphysema on their computed tomography (CT) scan (vs. 26.2%, P=0.0003) (14). Indirect signs of the influence of the lung parenchyma’s quality can also be assumed considering that patients with PAL tend to have limited lung function with significantly lower FEV1 and lower FEV1 to forced vital capacity (FVC) ratio (12,14,21). Furthermore, the rate of sarcopenia was significantly higher in the PAL cohort (48.8% vs. 21.5%, P<0.001), which might pose another risk factor, although only data for 311 patients of our cohort was available. Nevertheless, it remains up to discussion and further investigation if sarcopenia represents a confounder, as literature shows COPD being associated with sarcopenia (22). On the other hand, a dismal nutritional status, which poses a risk factor for complications, represents an influenceable condition amenable to preoperative optimization with recently proposed prehabilitation programs (23).
For risk prediction Brunelli et al. published a logistic risk model, an aggregate risk score (dividing the patients into class A–D with the risk ranging from 1.4% to 29% in the derivation set) and a digital PAL risk score. In the logistic risk model only FEV1 and pleural adhesions were of significant predictive value. In the aggregate risk score age >65 years, pleural adhesions, FEV1 <80% and body mass index <25.5 kg/m2 and in the digital PAL risk score mean air leak flow (measured by digital drainage systems) and mean differential pleural pressure were of significance (24). Comparing our findings from the risk analysis, it followed most of the proposed predictors of PAL in Brunelli’s aggregate risk score: age >65 years (66.2 vs. 63.5 years, P=0.022), pleural adhesions (not available), FEV1 <80% (74.4% vs. 82.5%, P<0.001) and body mass index <25.5 kg/m2 (24.0 vs. 25.5 kg/m2, P<0.001). Interestingly, for the BMI the decisive factor seems to be patients’ size, rather than weight, as the PAL cohort was significantly taller (172.6 vs. 169.9 cm, P=0.003), but not significantly lighter (71.7 vs. 73.8 kg, P=0.138), which might be explained by the above-mentioned hypothesis that an overdistention of the remaining lung is responsible for PAL (1). Because pleural adhesions are not documented in our cohort and since it is a rather subjective parameter, which cannot be sufficiently assessed preoperatively for risk assessment, we established our own risk prediction model. This was based on the 957 included patients. Accordingly, the odds of a patient developing a PAL after VATS resection can be calculated as follows: odds = econstant +0.022× age +1.016× sex (male =1) +0.007× size (cm) −0.110× BMI +0.699× COPD (yes =1) +0.501× resection involving right upper lobe (yes=1). Nevertheless, this model requires external validation to proof its discrimination and accuracy.
The mentioned risk factors are also confirmed by a systematic review and meta-analysis by Attaar et al., which found 17 significant risk factors in 37 included studies. The strongest associations with PAL were reported for FEV1% (mean difference =8.84), smoking history (OR 2.05), major anatomic lung resection (OR 2.82) and pleural adhesions (OR 1.94), but also risk factors such as age (OR 2.30), male sex (OR 1.68), COPD (OR 2.27) or upper resection (OR 1.34) proved to be of significant impact. However, as mentioned by the authors, all of the included studies were of low or very low quality of evidence (25).
Various studies have shown that PAL is an important risk factor for readmission after discharge from hospital following thoracic surgery (14,21,26,27). Readmission rate after or while still suffering from PAL range from 2.2% to 24.6%, depending on the study setting. Looking at a primary direct comparison between patient cohorts with and without PAL, patients with PAL are also at a significantly higher risk of developing further complications, which could also be seen in Liang et al.’s study showing an average of 1.26 complications in the PAL group in relation to 0.42 in the control group (P<0.05). These complications are manifold, but PAL also increases the risk for more severe morbidity with 73.8% (vs. 13.2%) developing a grade II and 26.1% (vs. 4.6%) a grade III or higher complication according to the Ottawa TM&M grades (14,27,28).
Attaar et al. and Liang et al. describe a higher rate of postoperative empyema as a pleural complication in their PAL cohorts than in their control groups. Attaar et al.’s data shows an empyema rate of 4.8% vs. 0.3% (P<0.001). Liang et al. reported an empyema rate of 11.8% in the group with severe PAL (requiring a major intervention or resulting in organ failure/death), making it, together with pneumonia, the most common complication (14,21). Empyema is often associated with pneumonia, with literature showing parapneumonic effusions in up to 40% of hospitalized pneumonia patients (7). Regarding postoperative differences in our own cohort, the PAL group naturally required their chest drains for a prolonged period before the first attempt of removal was possible (14.2 vs. 4.4 days, P<0.001). Most likely due to the prolonged chest drain duration and the active air fistula into the pleural cavity, the rate of postoperative infections was significantly elevated in the PAL cohort. Almost one-third of patients were affected (31.1% vs. 12.3%, P<0.001).
With an active air leak and therefore a connection between the alveolar space and the physiologically sterile pleura and about 11% of PAL patients developing pneumonia, it is of no surprise that the empyema rate in literature is significantly elevated (7). As a result, the readmission rate in the two mentioned cohorts was considerably higher with 15.2% (vs. 6.9%) and 24.6% (vs. 4.2%). Konstantinidis et al. and Seely et al. report similar readmission rates for PAL with 24% (vs. 9.2% in patients without PAL) and 17%, respectively. However, Seely et al. only compare a cohort with atrial fibrillation (2% readmission rate), representing their most common individual complication (26,27). This is also supported by Hasan et al.’s data, who report an empyema rate of 4% in their cohort with patients being discharged with a chest tube compared to 0% in their control group, which received autologous blood patch pleurodesis (ABPP) (29).
There are multiple hypotheses on reducing the occurrence of PAL, but only limited literature is available about the clinical impact measured through readmission. Hasan et al. investigated the effect of ABPP in a retrospective PAL cohort. In the no ABPP cohort the readmission rate of 19.7% was comparable with the already reported data. Still, the ABPP cohort showed a significantly lower readmission rate of 5.9% (P=0.02), although the postoperative in-hospital and after-discharge complication rates did not differ significantly (P=0.18; P=0.13) (29). In contrast to Hasan et al.’s data, Royer et al. report only a 3% readmission rate in their cohort of patients with a PAL being discharged with a chest tube and no case of empyema (30). Ponn et al. published results concurrent with Royer et al. with a readmission rate of 2.2% (i.e., one patient) in a postresection PAL cohort of 45 patients being discharged with a chest tube. The readmission was due to pneumonia and parapneumonic effusion (31).
Therefore, postoperative complications represent an important socio-economic factor. In our study 11.7% of the PAL cohort required a reoperation due to an air leak (vs. 0.2%, P<0.001). Furthermore, reoperated patients showed a significantly prolonged LOS with 28.36 days (vs. 10.13 days, P=0.021). Rising hospital costs put a strain on patient care and require a constant adaption of hospital financing, which is dependent on cash inflow and ways of cost reduction. Health care cost and its measurement remains a complex topic, cannot be generalized and varies heavily between countries, as many different financial and political policies exist (32-34). Nevertheless, extra costs for complications exist in every hospital system and need to be addressed to tackle excessive spending. Analyzing the available literature regarding the direct cost comparison of patient cohorts with and without PAL, PAL increases the overall cost of care (16-18,20,35,36). This cost increase is due to a prolonged postoperative LOS because of a higher rate of associated complications, chest drain duration, readmission, or reoperation, leading to a multiplication of fixed hospital costs, which is the same for every patient, and additional costs in the form of pharmaceutical treatment or interventions (16-18,20,36,37). Reported daily hospital costs ranged from 148 to 632.49 €, considering that most publications only publish total cost (15-18). Also, in our institutional cohort PAL necessitated a significantly longer LOS with 19.8 days (vs. 9.3 days, P<0.001). Brunelli et al. followed patients until hospital discharge and in a 90-day postoperative analysis showing higher treatment costs in PAL with total postoperative costs of 5,939.8 $ and 18,340 € in comparison to 4,381.7 $ and 13,102 € in the cohort without PAL (P=0.001; P<0.0001). The difference in costs between the two studies is explained by the 90-day follow-up, which covers readmission, outpatient department, medication, radiological imaging, and emergency department visits and therefore provides a more realistic measurement of additional expenses. In patients with PAL, who were readmitted, the costs of 27,671 € was 67% higher than in PAL patients without readmission (16,552 €, P<0.0001). Even when excluding patients with other associated cardiopulmonary complications, Brunelli et al. saw a significant cost increase in the PAL cohort with 15,016 € in comparison to 12,108 € (P=0.041) (16,17). Varela et al. present estimated excess total costs for PAL in their hospital (Salamanca, Spain) at 39.437 € (~1,878 € per patient,) solely calculated through the prolonged stay (18). In a Japanese cohort, Yotsukura et al. report 32% higher cost of hospital care for patients with PAL (19.176 vs. 14.490 $, P<0.001); also associated with a prolonged LOS (15.5 vs. 4.6 days, P<0.001) and a higher rate of other complications (16.5% vs. 1.8%, P<0.001) (20).
In US-American cohorts PAL is associated with significantly higher hospital charges with Wood et al. calculating with 98,809 $ (vs. 88,840 $, P<0.001) on average for their PAL cohort. Stratifying the study population according to their LOS, the authors found that costs increased significantly with a prolonged hospital stay. Patients with a LOS under 7 days led to total hospital charges of 64.682 $, with 7 to 10 days to 82,954 $ and 7 days or more to 149,475 $ (P<0.001) (36). Geller et al. report PAL as their most frequent major pulmonary complication, affecting 8.6% of their cohort and accounting for 13.8% of all complications. The associated 90-day cost increase for PAL was 21.8% (P<0.001) in comparison to an average 57.4% increase for major pulmonary complications in general (P<0.001) (35).
In a Chinese cohort Xu et al. analyzed the difference between a fissureless technique lobectomy using staplers and an electrocautery and suture division. The fissureless technique showed a lower PAL rate (11% vs. 30%, P=0.02), but, in contrast to the mentioned data above, higher perioperative costs (7,459.07 vs. 6,463.28 €, P<0.01), while having a comparable postoperative LOS (7.62 vs. 7.63 days, P=0.16). A limitation of this study is that the advantage of a lower PAL rate cannot be fully grasped, because even if patients got their chest tube removed on the first postoperative day, they still were kept in-hospital for about 1 week for observation and therefore negating possible potential savings through a shorter LOS (38). Although the study by Fung et al. does not thematize PAL after surgical resection, the publication shows a cost breakdown of PAL in connection with recurrent primary spontaneous pneumothorax. In case of recurrence, the total recurrence treatment costs amounted to an additional 1,353 € per patient, raising the overall management and complication costs per patient to 1,501 € in the VATS cohort and 2,233 € in the chest tube cohort (vs. 1,360 € and 1,247 € per patient for primary treatment) (15) Above mentioned publications by Fung et al. [2021], Brunelli et al. [2020], Brunelli et al. [2018] and Varela et al. [2005] have been used as a basis for estimation of estimated treatment costs for our cohort (15-18). As these publications stem from various health care systems with different economical structures and policies, the costs vary tremendously. To minimize confounders estimated cost was solely calculated using LOS data. For PAL patients, costs were significantly higher, ranging from 2,888.2 to 12,342.8 € in comparison to 1,370.5–5,856.8 € for the non-PAL cohort (P<0.001). When stratifying for reoperation during the stay for an air leak the cohort with reoperation had significantly higher estimated mean costs with 4,196.86 to 17,935.61 € (vs. 1,494.28 to 6,385.94, P=0.020).
For more detailed prospective studies, costs have to follow a distinct definition and should be determined in each healthcare system individually.
Limitations of our study are its retrospective design and cost estimation based on published data from different centers. Our proposed preoperative risk prediction model was not externally validated yet.
Conclusions
PAL still represents a common and rather expensive complication of thoracic surgery without widely accepted guidelines for management. Available guidelines tend to focus on spontaneous pneumothoraces rather than on postoperative air leaks, leading to various treatment options (39). The surgical technique is the only measure to reduce the PAL rate, as other risk factors, such as COPD, affected lobe or patient physique, cannot be altered. Whether other risk factors, like sarcopenia, can be influenced preoperatively, needs to be investigated further. If PAL occurs postoperatively, various treatment options with inconclusive results are reported in the literature, with in-hospital ABPP and discharge from hospital with chest drain being more common (29-31). Further investigation and high-volume prospective studies regarding treatment options and risk factors, such as described by Attaar et al., for PAL have to be undertaken to establish evidence-based guidelines and to be able to reduce the LOS and readmission rates (25). This is of utmost importance as most PALs in our cohort (88.3%) do not require surgical intervention and the excessive LOS, with or without readmission, remains the main matter of expense. Nevertheless, if patients have to undergo reoperation for PAL costs not only arise for re-do surgery, but also for a prolonged LOS in comparison to patients with a successful conservative management.
Acknowledgments
Funding: None.
Footnote
Provenance and Peer Review: This article was commissioned by the editorial office, Journal of Thoracic Disease, for the series “Prolonged Air Leak after Lung Surgery: Prediction, Prevention and Management”. The article has undergone external peer review.
Reporting Checklist: The authors have completed the STROBE reporting checklist. Available at https://jtd.amegroups.com/article/view/10.21037/jtd-21-2011/rc
Data Sharing Statement: Available at https://jtd.amegroups.com/article/view/10.21037/jtd-21-2011/dss
Conflicts of Interest: All authors have completed the ICMJE uniform disclosure form (available at https://jtd.amegroups.com/article/view/10.21037/jtd-21-2011/coif). The special series “Prolonged Air Leak after Lung Surgery: Prediction, Prevention and Management” was sponsored by Bard Limited. Bard Limited has no interference on the contents of the special series. AF served as the unpaid Guest Editor of the series. The authors have no other conflicts of interest to declare.
Ethical Statement: The authors are accountable for all aspects of the work in ensuring that questions related to the accuracy or integrity of any part of the work are appropriately investigated and resolved. The study was conducted in accordance with the Declaration of Helsinki (as revised in 2013). The study was approved by the ethics committee of the Medical University of Innsbruck (registration number: AN5163 327/4.17 382/5.2) and individual consent for this retrospective analysis was waived.
Open Access Statement: This is an Open Access article distributed in accordance with the Creative Commons Attribution-NonCommercial-NoDerivs 4.0 International License (CC BY-NC-ND 4.0), which permits the non-commercial replication and distribution of the article with the strict proviso that no changes or edits are made and the original work is properly cited (including links to both the formal publication through the relevant DOI and the license). See: https://creativecommons.org/licenses/by-nc-nd/4.0/.
References
- Pompili C, Miserocchi G. Air leak after lung resection: pathophysiology and patients' implications. J Thorac Dis 2016;8:S46-54. [Crossref] [PubMed]
- Brunelli A, Varela G, Refai M, et al. A scoring system to predict the risk of prolonged air leak after lobectomy. Ann Thorac Surg 2010;90:204-9. [Crossref] [PubMed]
- Cerfolio RJ, Bass CS, Pask AH, et al. Predictors and treatment of persistent air leaks. Ann Thorac Surg 2002;73:1727-30; discussion 1730-1. [Crossref] [PubMed]
- Ziarnik E, Grogan EL. Postlobectomy Early Complications. Thorac Surg Clin 2015;25:355-64. [Crossref] [PubMed]
- Abolhoda A, Liu D, Brooks A, et al. Prolonged air leak following radical upper lobectomy: an analysis of incidence and possible risk factors. Chest 1998;113:1507-10. [Crossref] [PubMed]
- DeCamp MM, Blackstone EH, Naunheim KS, et al. Patient and surgical factors influencing air leak after lung volume reduction surgery: lessons learned from the National Emphysema Treatment Trial. Ann Thorac Surg 2006;82:197-206; discussion 206-7. [Crossref] [PubMed]
- Light RW. Parapneumonic effusions and empyema. Proc Am Thorac Soc 2006;3:75-80. [Crossref] [PubMed]
- Liberman M, Muzikansky A, Wright CD, et al. Incidence and risk factors of persistent air leak after major pulmonary resection and use of chemical pleurodesis. Ann Thorac Surg 2010;89:891-7; discussion 897-8. [Crossref] [PubMed]
- Salati M, Brunelli A, Xiumè F, et al. Does fast-tracking increase the readmission rate after pulmonary resection? A case-matched study. Eur J Cardiothorac Surg 2012;41:1083-7; discussion 1087. [Crossref] [PubMed]
- Derstine BA, Holcombe SA, Ross BE, et al. Skeletal muscle cutoff values for sarcopenia diagnosis using T10 to L5 measurements in a healthy US population. Sci Rep 2018;8:11369. [Crossref] [PubMed]
- Hansen HJ, Petersen RH, Christensen M. Video-assisted thoracoscopic surgery (VATS) lobectomy using a standardized anterior approach. Surg Endosc 2011;25:1263-9. [Crossref] [PubMed]
- Pan H, Chang R, Zhou Y, et al. Risk factors associated with prolonged air leak after video-assisted thoracic surgery pulmonary resection: a predictive model and meta-analysis. Ann Transl Med 2019;7:103. [Crossref] [PubMed]
- Brunelli A, Monteverde M, Borri A, et al. Predictors of prolonged air leak after pulmonary lobectomy. Ann Thorac Surg 2004;77:1205-10; discussion 1210. [Crossref] [PubMed]
- Liang S, Ivanovic J, Gilbert S, et al. Quantifying the incidence and impact of postoperative prolonged alveolar air leak after pulmonary resection. J Thorac Cardiovasc Surg 2013;145:948-54. [Crossref] [PubMed]
- Fung S, Alexander A, Ashmawy H, et al. Socioeconomic Impact of Recurrent Primary Spontaneous Pneumothorax: Should Video-Assisted Thoracoscopic Surgery Be Considered at First Episode of Primary Spontaneous Pneumothorax? Healthcare (Basel) 2021;9:1236. [Crossref] [PubMed]
- Brunelli A, Chapman K, Pompili C, et al. Ninety-day hospital costs associated with prolonged air leak following lung resection. Interact Cardiovasc Thorac Surg 2020;31:507-12. [Crossref] [PubMed]
- Brunelli A, Pompili C, Dinesh P, et al. Financial validation of the European Society of Thoracic Surgeons risk score predicting prolonged air leak after video-assisted thoracic surgery lobectomy. J Thorac Cardiovasc Surg 2018;156:1224-30. [Crossref] [PubMed]
- Varela G, Jiménez MF, Novoa N, et al. Estimating hospital costs attributable to prolonged air leak in pulmonary lobectomy. Eur J Cardiothorac Surg 2005;27:329-33. [Crossref] [PubMed]
- Miserocchi G, Beretta E, Rivolta I. Respiratory mechanics and fluid dynamics after lung resection surgery. Thorac Surg Clin 2010;20:345-57. [Crossref] [PubMed]
- Yotsukura M, Okubo Y, Yoshida Y, et al. Predictive factors and economic impact of prolonged air leak after pulmonary resection. Gen Thorac Cardiovasc Surg 2022;70:44-51. [Crossref] [PubMed]
- Attaar A, Luketich JD, Schuchert MJ, et al. Prolonged Air Leak After Pulmonary Resection Increases Risk of Noncardiac Complications, Readmission, and Delayed Hospital Discharge: A Propensity Score-adjusted Analysis. Ann Surg 2021;273:163-72. [Crossref] [PubMed]
- Benz E, Trajanoska K, Lahousse L, et al. Sarcopenia in COPD: a systematic review and meta-analysis. Eur Respir Rev 2019;28:190049. [Crossref] [PubMed]
- Voorn MJJ, Beukers K, Trepels CMM, et al. Associations between pretreatment nutritional assessments and treatment complications in patients with stage I-III non-small cell lung cancer: A systematic review. Clin Nutr ESPEN 2022;47:152-62. [Crossref] [PubMed]
- Brunelli A, Cassivi SD, Halgren L. Risk factors for prolonged air leak after pulmonary resection. Thorac Surg Clin 2010;20:359-64. [Crossref] [PubMed]
- Attaar A, Tam V, Nason KS. Risk Factors for Prolonged Air Leak After Pulmonary Resection: A Systematic Review and Meta-analysis. Ann Surg 2020;271:834-44. [Crossref] [PubMed]
- Konstantinidis K, Woodcock-Shaw J, Dinesh P, et al. Incidence and risk factors for 90-day hospital readmission following video-assisted thoracoscopic anatomical lung resection†. Eur J Cardiothorac Surg 2019;55:666-72. [Crossref] [PubMed]
- Seely AJ, Ivanovic J, Threader J, et al. Systematic classification of morbidity and mortality after thoracic surgery. Ann Thorac Surg 2010;90:936-42; discussion 942. [Crossref] [PubMed]
- Ivanovic J, Al-Hussaini A, Al-Shehab D, et al. Evaluating the reliability and reproducibility of the Ottawa Thoracic Morbidity and Mortality classification system. Ann Thorac Surg 2011;91:387-93. [Crossref] [PubMed]
- Hasan IS, Allen MS, Cassivi SD, et al. Autologous blood patch pleurodesis for prolonged postoperative air leaks. J Thorac Dis 2021;13:3347-58. [Crossref] [PubMed]
- Royer AM, Smith JS, Miller A, et al. Safety of Outpatient Chest Tube Management of Air Leaks After Pulmonary Resection. Am Surg 2015;81:760-3.
- Ponn RB, Silverman HJ, Federico JA. Outpatient chest tube management. Ann Thorac Surg 1997;64:1437-40. [Crossref] [PubMed]
- Sturmberg JP, Bircher J. Better and fulfilling healthcare at lower costs: The need to manage health systems as complex adaptive systems. F1000Res 2019;8:789. [Crossref] [PubMed]
- Culyer AJ. Cost containment in Europe. Health Care Financ Rev 1989;21-32.
- Negrini D, Kettle A, Sheppard L, et al. The cost of a hospital ward in Europe: is there a methodology available to accurately measure the costs? J Health Organ Manag 2004;18:195-206. [Crossref] [PubMed]
- Geller AD, Zheng H, Mathisen DJ, et al. Relative incremental costs of complications of lobectomy for stage I non-small cell lung cancer. J Thorac Cardiovasc Surg 2018;155:1804-11. [Crossref] [PubMed]
- Wood DE, Lauer LM, Layton A, et al. Prolonged length of stay associated with air leak following pulmonary resection has a negative impact on hospital margin. Clinicoecon Outcomes Res 2016;8:187-95. [Crossref] [PubMed]
- Lackey A, Mitchell JD. The cost of air leak: physicians' and patients' perspectives. Thorac Surg Clin 2010;20:407-11. [Crossref] [PubMed]
- Xu H, Zhang L. Dissection of Incomplete Fissure Using Electrocautery Is a Safe and Acceptable Method for Thoracoscopic Right Upper Lobectomy. Thorac Cardiovasc Surg 2019;67:227-31. [Crossref] [PubMed]
- Dugan KC, Laxmanan B, Murgu S, et al. Management of Persistent Air Leaks. Chest 2017;152:417-23. [Crossref] [PubMed]