This article has an erratum available at: http://dx.doi.org/10.21037/jtd-2022-22 the article has been update on 2022-12-28 at here.
The effects of hydrogen treatment in a cigarette smoke solution-induced chronic obstructive pulmonary disease-like changes in an animal model
Introduction
Chronic obstructive pulmonary disease (COPD) is a common inflammatory disease with high morbidity and mortality. It is the third most common cause of death globally, as estimated by World Health Organization (WHO) in 2019 (1). It was reported that 10% of adults over 40 suffered from this disease (2). COPD caused by harmful particles and gases, especially tobacco smoke, is characterized by chronically abnormal respiratory responses, such as airway inflammation and emphysema (3). The Global Initiative for Chronic Obstructive Lung Disease (GOLD) science committee suggests that patients with COPD exacerbations should use systemic steroids, antibiotics, oxygen (O2) supplementation, and other protective lung strategies (4). Currently, these treatments are intended to relieve the symptoms or prevent the exacerbation of the disease. None of these interventions reverses the damage to the lung. Therefore, there is still a need for novel therapeutics to prevent and treat COPD.
A recent review of the literature indicated that hydrogen (H2) could be a valuable treatment for many diseases (5). H2 can serve as a scavenger of free radicals such as hydroxyl radicals (OH) and thus exert anti-oxidative (6) and anti-inflammatory (7) effects. Moreover, the signaling-regulating (8) and anti-apoptotic (9) effects of H2 play a significant role in a wider range of diseases. Hydrogen has been demonstrated to treat COPD, cerebral ischemia, metabolic syndrome, arthritis and side effects of chemotherapy. Many pre-clinical studies have found that inhalation of H2 may have the potential to be a therapy for COPD. Liu et al. in 2017 (10) and Lu et al. in 2018 (11) have found positive effects of inhaling hydrogen gas in cigarette smoke (CS)-induced animal models. This paper examines the effects of hydrogen gas inhalation, administered by using the advanced technology of Oxy-Hydrogen Generator, on COPD-like injury in a mouse model. To ensure that the mice received the same level of induction, this study applied cigarette smoke solution (CSS) by intraperitoneal (IP) injection. Zhang et al. in 2013 (12) have demonstrated the IP injection of CS extract induced emphysema, and He et al. in 2015 (13) have suggested that both CS exposure and CSS IP injection modes could have caused similar levels of emphysema. It is hypothesized that inhaled hydrogen gas may reduce the mortality rate, weight loss and other lung damage associated with CSS-induced COPD-like injury in this mouse model. In addition, the hydrogen treatment was started on the first day of the experiment to demonstrate the protective effect of molecular hydrogen in COPD-like injury. We present the following article in accordance with the ARRIVE reporting checklist (available at https://jtd.amegroups.com/article/view/10.21037/jtd-22-324/rc).
Methods
Animals
Thirty female BALB/c mice (6 weeks old) were purchased from BioLasco Taiwan Co., Ltd. And quarantined for one week. Animals were group-housed (4–5 mice in one cage) in the Taiwan Mouse Clinic, at a constant temperature (18–20 ℃) and humidity (30–70%) with 12 h/12 h light/dark cycles. Animals had free access to rodent pellet food (PicoLab® Rodent Diet 20) and drank water ad libitum. All animal study protocol was reviewed and approved by the Institutional Animal Care and Use Committee of Development Center for Biotechnology (IACUC-2020-R501-043), in compliance with Guideline for the Care and Use of Laboratory Animals, Council of Agriculture, Executive Yuan, Taipei.
Preparation of CSS
CSS was prepared, with modifications, from a previously described protocol (12). Cigarettes (brand: New Paradise; tar: 10 mg; nicotine: 0.8 mg) were burned, and the smoke was collected via peristaltic pump (35 mL/min). The smoke was filtered to remove particles and delivered into a vessel with phosphate-buffered saline (10 mL). The average nicotine concentration in the CSS was 114 µg/mL as measured by high-performance liquid chromatography (HPLC).
Oxy-Hydrogen Generator
The Oxy-Hydrogen Generator (HOHO Biotech Co., LTD., Taipei) produces hydrogen and oxygen gases from reverse osmosis of water. The output gas was 42% H2 and 21% O2 and delivered with 43% air through a Y-shaped tube at a flow of 0.9 L/min to the group of mice housed in an inhalation box. Mice body weights were measured twice weekly.
Treatment regimen of CSS in mice
Figure 1 shows the study flow. Mice were randomly divided into three groups: Control group (n=8), COPD group (n=10) and COPD + H2/O2 group (n=12). The average body weight of the mice was 21.6±0.3 grams. Mice were treated with 0.48 mL of double-distilled water (Control group) or CSS (COPD induction groups) twice weekly via IP injection for 39 days (Figure 1). The COPD + H2/O2 group was also placed into an inhalation box connected to the Oxy-Hydrogen Generator. The H2/O2 dosing regime was 75 minutes, twice daily, with four hours in between, for 63 days.

Neutrophil elastase (NE) activity
Mouse thorax fur was removed prior to fluorescence imaging analyses. NE 680 FAST (NEV11169, PerkinElmer) was used as an imaging agent and administered (100 µL) via tail vein injection. Mice were anesthetized with an inhalation anesthetic (isoflurane) and placed under the in vivo X-treme imaging system to detect fluorescent signals. Image analyses were performed with molecular imaging software (Bruker). The region of interest (ROI) was drawn in the thorax, and the fluorescence intensity within the ROI was calculated.
Lung histological evaluations
Four mice in the control group, 4 in the COPD, and 6 in the COPD + H2/O2 group with higher fluorescence intensity levels from the X-treme imaging system were sacrificed. The lungs were preserved in 10% neutral buffered formalin (NBF), trimmed, embedded in paraffin, sectioned, stained with hematoxylin and eosin (H&E) and Periodic Acid-Schiff (PAS) stains, and examined microscopically with an optical microscope (Leica DM2700M, USA) by a veterinary pathologist. (BioLASCO Taiwan Co., Ltd., Pathology & Toxicology Laboratory).
Lung sections were stained with H&E to score lesions and with PAS to determine goblet cell density. According to Shackelford et al. in 2002 (14), the lesion was scored as follows: 0, normal; 1, minimal, <1%; 2, slight, 1–25%; 3, moderate, 26–50%; 4, moderately severe, 51–75%; 5, severe/high, 76–100%. Mean linear intercept (MLI), assessed by ImageJ software, was calculated by the total length of alveoli divided by the number of alveoli per field under light microscopy. This value gives a measure of the enlargement and destruction of alveoli.
Statistical analysis
All data were presented as mean ± standard deviation (SD). Statistical comparison among groups was carried out by two-way analysis of variance (ANOVA) (SPSS, Ver. 22.0) and Bonferroni’s test. The histopathologic scores were analyzed by Wilcoxon ranked sum test. Statistical significance was set at P<0.05.
Results
The effect of H2/O2 inhalation on survival rates and body weight
The experiment lasted 67 days: 39 days for CSS induction, 24 days for the development of COPD-like pathological changes, and a final 4 days for analyses (Figure 1). During the CSS-induction period, the COPD group had two deaths that occurred on the 8th and 15th days, respectively, with an 80% survival rate (Figure 2). The COPD + H2/O2 group had a 100% survival rate. This preliminary result suggests that H2/O2 inhalation may reduce the risk of death from COPD-like injury in this model.
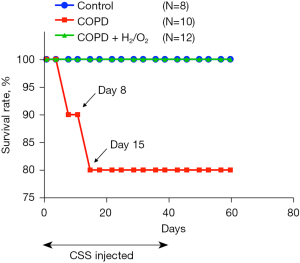
Hydrogen inhalation did not prevent the decrease in body weight caused by CSS (Figure 3). There was a slight decrease in body weight gain in COPD and COPD + H2/O2 groups during the CSS injection period.
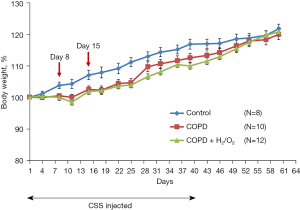
The effect of H2/O2 inhalation on NE activity
The level of NE680 Fast fluorescence reflects NE enzyme activity, which plays a major role in pulmonary inflammation in COPD. NE activity in the lungs of the CSS-induced COPD-like injury in mice was detected after the end of the treatment (day 64). Figure 4A shows representative images of NE activity. Figure 4B is a dot plot showing the fluorescence intensity of animals in each group. The mean levels of NE680 Fast fluorescence intensity in control, COPD, and COPD + H2/O2 groups were 163, 182.1, and 168 (×108 P/S/mm2), respectively (Figure 4B). There was no statistically significant difference in the mean levels of fluorescence intensity among the three groups.
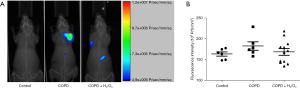
H2/O2 inhalation reduces CSS-induced inflammation and emphysema
Figure 5A shows histopathologic sections in the three groups. The lung section in the COPD group had alveolar wall damage, consistent with inflammation and emphysema. The MLI of the lung in the COPD + H2/O2 group (36.254±7.487) was significantly decreased compared to the COPD group (52.068±16.473) (P<0.05, Figure 5B).
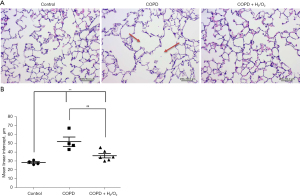
The total histopathological lesion scores (inflammation and emphysema) were significantly higher in the COPD group compared to the control group (P<0.05), and a significantly lower lesion score (3.17±0.98) was noted in the COPD + H2/O2 group compared to the COPD group (5.25±0.96) (P<0.05) (Table 1).
Table 1
Measurements | Groups | ||
---|---|---|---|
Control (mean ± SD) |
COPD (mean ± SD) |
COPD + H2/O2 (mean ± SD) |
|
Inflammation | 1.75±0.50 | 2.50±0.58 | 1.67±0.52# |
Emphysema | 0.75±0.96 | 2.75±0.50* | 1.50±0.55## |
Total histological score | 2.50±1.29 | 5.25±0.96* | 3.17±0.98# |
The mean lesion scores of the H&E-stained lung tissues for each group were presented. *, P<0.05 (significant difference between control and other groups); #, P<0.05; ##, P<0.01 (significant difference between the COPD and the COPD + H2/O2 groups). n=4 for control and COPD group, and n=6 for COPD + H2/O2 group. H2/O2, hydrogen-oxygen; CSS, cigarette smoke solution; COPD, chronic obstructive pulmonary disease; SD, standard deviation; H&E, hematoxylin and eosin stain.
H2/O2 inhalation reduces CSS-induced goblet cell hypertrophy and hyperplasia of airway epithelium
The PAS-stained lung sections showed histopathological changes in the COPD-like injury mice, with goblet cell hypertrophy and hyperplasia of airway epithelium (Figure 6A). The lesion score was significantly greater in the COPD group (1.75) than in the control group (0.25) (P<0.01). There was no statistically significant difference between the COPD and the COPD + H2/O2 groups (Figure 6B).
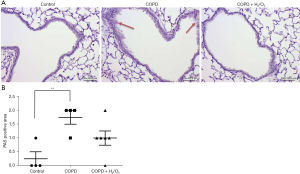
Discussion
COPD is recognized as a preventable, treatable but irreversible respiratory disease mainly triggered by cigarette smoking (15). The yearly increasing mortality rate makes COPD treatment a significant worldwide issue (16). A typical characteristic of COPD is constant airflow limitation developed from obstructive bronchiolitis and emphysema (17). Patients may suffer from dyspnea and chronic cough, which lead to comorbidities and exacerbated conditions (18). To our knowledge, there is still no therapeutic agent that can completely reverse COPD. Therefore, the searching for a novel preventive treatment to reduce the risk of COPD remains an important and urgent medical need.
Hydrogen has been found to have anti-oxidative and anti-inflammatory effects. Moreover, the safety of H2 usage in diving has been established since 1994 (19), suggesting that H2 is likely to be safer and thus become a more widely used therapeutics. Many of the properties of hydrogen support the benefits of medical treatment. For example, H2 can penetrate bio-membranes and reach cytosol and organelles because of its small molecular weight. Furthermore, the compatibility of H2 with other tissues is also higher than other oxidant scavengers (20). The potential effectiveness of H2 inhalation in preventing COPD-like injuries, as demonstrated in this study, adds to the importance of pursuing its therapeutic benefits.
Our results are similar to two studies which analyzed the benefits of inhaling hydrogen on cigarette smoking-induced COPD disease in animal models (10,11). However, two main differences are worth noting. Firstly, the two studies used CS inhaled by animals to induce COPD, while CSS was applied via injection in our study. It has been observed that sometimes mice hide in the corner of the chamber to escape from the toxic smoke when CS is used as an inducer. Moreover, the individual differences in breath frequency and the amount of inhaled gas in each breath might increase the variability of dose administration during CS induction. However, He et al. in 2015 (13) suggested that both CS exposure and CSS IP injection could have the same effectiveness and similar values of emphysema. We selected the IP injection application of CSS in order to reduce the variability of the amount of CS delivered during the study. A second difference between our approach and that used in the above-mentioned two studies is the hydrogen generating technology. The hydrogen gas used in this study was provided via the Oxy-Hydrogen Generator containing a proton exchange membrane, the latest technology in generating hydrogen gas. By avoiding direct contact with metals, this equipment can produce pure hydrogen without toxic by-products. Furthermore, hydrogen could be generated at a lower temperature, allowing a safer environment when applied to the experiment.
To evaluate the preventive effect of H2 on the risk of mortality caused by COPD-like injury, the survival rate of mice was calculated in this study. The 80% survival rate in the COPD group demonstrated the toxicity and lethal effects of CSS on the mice. H2/O2 inhalation might attenuate this effect and result in a 100% survival rate. However, in future studies, autopsies, to characterize the cause of death, need to be included to document the contribution of H2/O2 therapy in increasing the survival rate. Moreover, the small number of events also makes it challenging to obtain a statistically robust conclusion for the efficacy of the Oxy-Hydrogen Generator on survival benefit.
One recent study demonstrated the weight loss in animal models from cigarette smoking treatment and ameliorating effects of the H2 inhalation (10). However, in this study, only a slight decrease in weight gain was found in groups when treated with CSS IP injection, and no protective effects of H2 inhalation were observed. This result is consistent with the finding made by Ardite et al. (21), which strongly suggested that the weight gain reduction of the COPD animal model may be due to the toxicity of cigarettes and appears to be independent of the changes and treatment in the lung.
Pulmonary function analysis is a standard tool for evaluating lung-related diseases, including COPD. Taking forced vital capacity (FVC) value for example, COPD patients usually have a lower FVC value than healthy people. Thus, pulmonary function analysis is also applied in animal models to develop novel medicines and devices for lung treatment. However, the different ways of measuring lung functions in human and animal models are of concern. The values measured from humans focus on active respiration values, while only passive respiration values can be detected from animals. This defect can be seen in Lu et al. in 2018 (11), where an increase in FVC value was found in the CS-induced mice. This result may be because a forced expiration maneuver was used, causing CS-induced mice with airspace enlargement to have an increase in FVC value. Based on the above consideration, we did not measure pulmonary function in this study.
NE 680 Fast is a novel technology used in an animal model to detect neutrophil-mediated inflammation. It was first used in a mice lung injury experiment in 2011 to image and quantify NE activity (22). COPD is well known to have lung inflammation associated with NE; therefore, it is reasonable to use NE 680 Fast as a pre-analysis before the histopathology study. It is generally believed that healthy animals also have chronic inflammation because of oxidative effects inside the body. In the study, mice in the control group had mean fluorescence intensity of 163 (×108 P/S/mm2). Although the result in this study shows that there is no significant difference between the three groups on mean values of the fluorescence intensity, more mice with lower fluorescence intensity than the mean value of control were found in the COPD + H2/O2 group, suggesting possible inflammatory protection of H2 inhalation from CSS-induced COPD-like injury. Interestingly, some mice in the COPD + H2/O2 group even had fluorescence intensity lower than mice in the control group. This result proposes a possibility that H2/O2 inhalation may not only reduce the damage from CSS-induced COPD-like injury but also reduce inflammation in a healthy mice body.
Histopathologic changes can be used to determine the seriousness of COPD development. Inflammatory and emphysema, two major lesion scores (23) in COPD development, were analyzed in this study. A well-acceptable mechanism of emphysema is the destruction of lung elastin induced by the imbalance of protease and antiprotease activity (24), leading to permanent hyperextension and loss of elastic recoil of alveolar walls (2,25). Hence, in the histopathological analysis, alveolar wall destruction and airspace enlargement can be seen in emphysema. In this study, the above emphysema features were shown in histopathologic pictures captured under a light microscope in the COPD group. Less alveolar wall damage was found in the COPD + H2/O2 group, indicating a preventive effect of H2 in the development of emphysema-like changes. This result is consistent with the lesion scores reported by veterinary pathologists with significantly higher emphysema scores in the COPD group, whereas lower scores in the COPD + H2/O2 group. In addition, the measurement of MLI based on evaluating quantitatively histological changes (26) can objectively reflect the enlargement of alveolar spaces (25,27) associated with the development of emphysema in the lung section. A higher value of MLI indicates more severe emphysema, which can be seen in the COPD group in this study. In contrast, the COPD + H2/O2 group had a significant lower MLI, confirming the effectiveness of H2 in reducing emphysema-like changes.
One symptom of COPD in patients is airflow obstruction caused by mucus hypersecretion (28). On a molecular scale, goblet cell hyperplasia leads to the hypersecretion of the mucus (29). Thus, observing hyperplasia of goblet cells in the lung section is a common way to understand the development of COPD. In this study, a significant increase of goblet cells in the COPD group was found in both visual observation and PAS score. However, the COPD + H2/O2 group showed an insignificant decrease in the PAS score compared to that of the COPD group. In a study by Ning et al., hydrogen-rich saline injection significantly attenuated CS inhalation-induced mucus hypersecretion (30). It could be that direct CS exposure to goblet cells triggers more mucus production. And further studies are needed to confirm the optimal route and dosage of molecular hydrogen for mucus hypersecretion.
Conclusions
In conclusion, H2/O2 inhalation can potentially reduce the risk of developing CSS-induced COPD-like injury. This potential is particularly strong for the development of emphysema-like changes but less clear for the reduction of mucus secretion. These positive results could be attributable to the anti-inflammatory activity of hydrogen. Given the safety of hydrogen therapy, future clinical trials should be considered to confirm the safety and efficacy of H2/O2 in, for example, COPD patients.
Acknowledgments
Funding: This study was supported by HOHO Biotech Co., Ltd.
Footnote
Reporting Checklist: The authors have completed the ARRIVE reporting checklist. Available at https://jtd.amegroups.com/article/view/10.21037/jtd-22-324/rc
Data Sharing Statement: Available at https://jtd.amegroups.com/article/view/10.21037/jtd-22-324/dss
Peer Review File: Available at https://jtd.amegroups.com/article/view/10.21037/jtd-22-324/prf
Conflicts of Interest: All authors have completed the ICMJE uniform disclosure form (available at https://jtd.amegroups.com/article/view/10.21037/jtd-22-324/coif). HJY, WHT, HMS, KYW, and FLD reports funding from HOHO Biotech Co., Ltd. HJY is the medical advisor of HOHO Biotech. KYW is the founder of HOHO Biotech. WHT is the employee of HOHO Biotech. HMS and FLD are board members of HOHO Biotech. HMS is the person in charge for IPC Intellectual Property Connections, INC. She declares that IPC Intellectual Property Connections, INC. has no relationship with HOHO and does not have any interest in the publication of the present article. The other authors have no conflicts of interest to disclose.
Ethical Statement:
Open Access Statement: This is an Open Access article distributed in accordance with the Creative Commons Attribution-NonCommercial-NoDerivs 4.0 International License (CC BY-NC-ND 4.0), which permits the non-commercial replication and distribution of the article with the strict proviso that no changes or edits are made and the original work is properly cited (including links to both the formal publication through the relevant DOI and the license). See: https://creativecommons.org/licenses/by-nc-nd/4.0/.
References
- WHO. The top 10 causes of death. World Health Organization, 2020. Available online: https://www.who.int/news-room/fact-sheets/detail/the-top-10-causes-of-death
- Barnes PJ. The cytokine network in asthma and chronic obstructive pulmonary disease. J Clin Invest 2008;118:3546-56. [Crossref] [PubMed]
- Hassett DJ, Borchers MT, Panos RJ. Chronic obstructive pulmonary disease (COPD): evaluation from clinical, immunological and bacterial pathogenesis perspectives. J Microbiol 2014;52:211-26. [Crossref] [PubMed]
- Halpin DMG, Criner GJ, Papi A, et al. Global Initiative for the Diagnosis, Management, and Prevention of Chronic Obstructive Lung Disease. The 2020 GOLD Science Committee Report on COVID-19 and Chronic Obstructive Pulmonary Disease. Am J Respir Crit Care Med 2021;203:24-36. [Crossref] [PubMed]
- Ohta S. Molecular hydrogen as a preventive and therapeutic medical gas: initiation, development and potential of hydrogen medicine. Pharmacol Ther 2014;144:1-11. [Crossref] [PubMed]
- Xie K, Yu Y, Pei Y, et al. Protective effects of hydrogen gas on murine polymicrobial sepsis via reducing oxidative stress and HMGB1 release. Shock 2010;34:90-7. [Crossref] [PubMed]
- Gharib B, Hanna S, Abdallahi OM, et al. Anti-inflammatory properties of molecular hydrogen: investigation on parasite-induced liver inflammation. C R Acad Sci III 2001;324:719-24. [Crossref] [PubMed]
- Itoh T, Hamada N, Terazawa R, et al. Molecular hydrogen inhibits lipopolysaccharide/interferon γ-induced nitric oxide production through modulation of signal transduction in macrophages. Biochem Biophys Res Commun 2011;411:143-9. [Crossref] [PubMed]
- Sun Q, Kang Z, Cai J, et al. Hydrogen-rich saline protects myocardium against ischemia/reperfusion injury in rats. Exp Biol Med (Maywood) 2009;234:1212-9. [Crossref] [PubMed]
- Liu X, Ma C, Wang X, et al. Hydrogen coadministration slows the development of COPD-like lung disease in a cigarette smoke-induced rat model. Int J Chron Obstruct Pulmon Dis 2017;12:1309-24. [Crossref] [PubMed]
- Lu W, Li D, Hu J, et al. Hydrogen gas inhalation protects against cigarette smoke-induced COPD development in mice. J Thorac Dis 2018;10:3232-43. [Crossref] [PubMed]
- Zhang Y, Cao J, Chen Y, et al. Intraperitoneal injection of cigarette smoke extract induced emphysema, and injury of cardiac and skeletal muscles in BALB/C mice. Exp Lung Res 2013;39:18-31. [Crossref] [PubMed]
- He ZH, Chen P, Chen Y, et al. Comparison between cigarette smoke-induced emphysema and cigarette smoke extract-induced emphysema. Tob Induc Dis 2015;13:6. [Crossref] [PubMed]
- Shackelford C, Long G, Wolf J, et al. Qualitative and quantitative analysis of nonneoplastic lesions in toxicology studies. Toxicol Pathol 2002;30:93-6. [Crossref] [PubMed]
- Vestbo J. COPD: definition and phenotypes. Clin Chest Med 2014;35:1-6. [Crossref] [PubMed]
- Patel AR, Patel AR, Singh S, et al. Global Initiative for Chronic Obstructive Lung Disease: The Changes Made. Cureus 2019;11:e4985. [Crossref] [PubMed]
- Menezes AM, Wehrmeister FC, Perez-Padilla R, et al. The PLATINO study: description of the distribution, stability, and mortality according to the Global Initiative for Chronic Obstructive Lung Disease classification from 2007 to 2017. Int J Chron Obstruct Pulmon Dis 2017;12:1491-501. [Crossref] [PubMed]
- Eisner MD, Anthonisen N, Coultas D, et al. An official American Thoracic Society public policy statement: Novel risk factors and the global burden of chronic obstructive pulmonary disease. Am J Respir Crit Care Med 2010;182:693-718. [Crossref] [PubMed]
- Abraini JH, Gardette-Chauffour MC, Martinez E, et al. Psychophysiological reactions in humans during an open sea dive to 500 m with a hydrogen-helium-oxygen mixture. J Appl Physiol (1985) 1994;76:1113-8. [Crossref] [PubMed]
- Wang ST, Bao C, He Y, et al. Hydrogen gas (XEN) inhalation ameliorates airway inflammation in asthma and COPD patients. QJM 2020;113:870-5. [Crossref] [PubMed]
- Ardite E, Peinado VI, Rabinovich RA, et al. Systemic effects of cigarette smoke exposure in the guinea pig. Respir Med 2006;100:1186-94. [Crossref] [PubMed]
- Kossodo S, Zhang J, Groves K, et al. Noninvasive in vivo quantification of neutrophil elastase activity in acute experimental mouse lung injury. Int J Mol Imaging 2011;2011:581406. [Crossref] [PubMed]
- Groneberg DA, Chung KF. Models of chronic obstructive pulmonary disease. Respir Res 2004;5:18. [Crossref] [PubMed]
- Takubo Y, Guerassimov A, Ghezzo H, et al. Alpha1-antitrypsin determines the pattern of emphysema and function in tobacco smoke-exposed mice: parallels with human disease. Am J Respir Crit Care Med 2002;166:1596-603. [Crossref] [PubMed]
- Kim WD, Eidelman DH, Izquierdo JL, et al. Centrilobular and panlobular emphysema in smokers. Two distinct morphologic and functional entities. Am Rev Respir Dis 1991;144:1385-90. [Crossref] [PubMed]
- Dunnill MS. Evaluation of a simple method of sampling the lung for quantitative histological analysis. Thorax 1964;19:443-8. [Crossref] [PubMed]
- Chung KF, Adcock IM. Multifaceted mechanisms in COPD: inflammation, immunity, and tissue repair and destruction. Eur Respir J 2008;31:1334-56. [Crossref] [PubMed]
- Innes AL, Woodruff PG, Ferrando RE, et al. Epithelial mucin stores are increased in the large airways of smokers with airflow obstruction. Chest 2006;130:1102-8. [Crossref] [PubMed]
- Aikawa T, Shimura S, Sasaki H, et al. Morphometric analysis of intraluminal mucus in airways in chronic obstructive pulmonary disease. Am Rev Respir Dis 1989;140:477-82. [Crossref] [PubMed]
- Ning Y, Shang Y, Huang H, et al. Attenuation of cigarette smoke-induced airway mucus production by hydrogen-rich saline in rats. PloS One 2013;8:e83429. [Crossref] [PubMed]