Analysis of systemic vascular resistance after cardiac surgery: a retrospective cohort study
Introduction
Cardiac surgeries are regularly performed worldwide. This remarkable development is attributable to advancements in cardiopulmonary bypass (CPB). However, cardiac surgery still presents high surgical mortality rates. Therefore, postoperative care should be provided with great caution, and hemodynamics should be considered.
After cardiac surgery, practitioners generally monitor patients for the development of hypotension, which can cause circulatory failure. However, hypertension caused by excessive constriction of peripheral blood vessels can cause peripheral circulatory failure. This illustrates the difficulty of monitoring peripheral circulation using blood pressure alone. Therefore, patient management must involve the careful consideration of the peripheral circulation, blood pressure measurements, intravascular volume, cardiac output, and vascular resistance. For example, when the blood pressure is high, vascular resistance is high, and cardiac output is low; therefore, vasodilators or volume administration may be necessary. Furthermore, when blood pressure is low and vascular resistance is low, catecholamines or other drugs should be used to increase peripheral vascular resistance. Therefore, systemic vascular resistance is extremely important and should always be considered after cardiac surgery because it is a parameter that directly affects blood pressure and peripheral circulation when the cardiac output and intravascular volume are the same. However, the factors that influence vascular resistance are not fully understood. These factors include sex, age, preoperative conditions, surgical technique, CPB type or duration, duration of surgery, and the amount of cardioplegia. The only way to maintain peripheral circulation is to follow the changes in vascular resistance (1-6).
We analyzed whether the systemic vascular resistance index (SVRI) differs according to the type of cardiac surgery, which may be one of the possible factors affecting vascular resistance. If the characteristics of the SVRI can be determined, then the quality of postoperative care for patients may improve and mortality rates after cardiac surgery may decrease. We present the following article in accordance with the STROBE reporting checklist (available at https://jtd.amegroups.com/article/view/10.21037/jtd-22-325/rc).
Methods
IRB/informed consent
This retrospective cohort study analyzed the data of adult patients who underwent cardiac surgery at Asahi General Hospital between April 2014 and August 2020. The inclusion criteria were as follows: (I) underwent cardiac surgery at our hospital, (II) Swan-Ganz data are traceable, and (III) no missing biometric data. The exclusion criteria were as follows: (I) bleeding of more than 300 mL/hour or cardiac tamponade which led to reoperation for hemostasis, (II) intra-aortic balloon pumping and/or extracorporeal membrane oxygenation were used, (III) pulmonary hypertension with systolic pulmonary artery pressure greater than half the systolic aortic pressure, (IV) reoperation cases, (V) or patients who abandoned treatment during surgery or within 12 hours after surgery. The study was conducted in accordance with the Declaration of Helsinki (as revised in 2013). The study was approved by the Institutional Committee Board of Asahi General Hospital (IRB number: 2020111725; date of approval: November 17, 2020). Because of the retrospective nature of the study, we used the opt-out approach to obtain informed consent from all participants.
Perioperative procedure/anesthetic management
All patients were anesthetized using a routine technique comprising midazolam and fentanyl for induction, rocuronium bromide for muscle relaxation, and sevoflurane, fentanyl, and propofol for maintenance. The right radial artery was used to measure blood pressure and collect blood for the blood gas analysis. A central venous catheter and a Swan-Ganz catheter (CCOmbo V catheters; Edwards Lifesciences Corporation, Irvine, CA, USA) were inserted through the right common carotid vein. We collected data regarding the cardiac output, cardiac index, mixed venous oxygen saturation, central venous pressure (CVP), pulmonary artery pressure, and SVRI. The SVRI was calculated as follows: SVRI = (mean blood pressure- mean CVP)/cardiac index × 79.92 (dyne·s·cm-5·m2). All data obtained using the Swan-Ganz catheter during the intraoperative and postoperative phases were analyzed using a Vigilance II monitor (model number VIG2; Edwards Lifesciences Corporation).
Patients were draped and placed in the supine or right lateral recumbent position. A mid-sternal incision or left thoracotomy was performed to access the surgical site. Heparin was administered preoperatively and intraoperatively to maintain the activated clotting time at more than 400 s.
CPB (total bypass) was used during all mitral valve (MV) and aortic valve (AV) surgeries, during almost all thoracic aorta (TA) surgeries, and during some coronary artery bypass surgeries (CABGs). It was established by ascending aorta perfusion and bicaval drainage through a mid-sternal incision. The bladder temperature usually decreased to 30 to 32 ℃; however, it was reduced to 20 ℃ during aortic surgery when circulation arrest was performed to preserve vital organs, especially the brain. The ascending aorta was clamped, and cardiac arrest was triggered by antegrade coronary perfusion with cardioplegia. Cardiac arrest was maintained by intermittent retrograde coronary perfusion with infusion of cardioplegic fluid from the coronary sinus. The cardioplegic solution consisted of St. Thomas solution and blood in a 1:1 ratio. After completing the surgical procedures, the ascending aorta was declamped, and the heartbeat resumed. After checking the cardiac function (by measuring parameters such as blood pressure and cardiac index) and circulating blood volume (by measuring CVP), hemostasis, and body temperature (more than 36 ℃), patients were weaned off CPB. The cardiac function was maintained by administering catecholamines, such as dopamine, dobutamine, norepinephrine, and (sometimes) epinephrine. If catecholamines were insufficient for maintaining the hemodynamics, then intra-aortic balloon pumping or percutaneous cardiopulmonary support was commenced; if mechanical support was not indicated, then protamine (1 mg per 100 units of heparin) was injected to reverse the effect of heparin.
CPB (partial bypass) was used for descending aortic surgery (part of TA surgery). CPB was established with femoral artery perfusion and femoral vein drainage, and the heart rate was maintained with natural cooling to lower the bladder temperature to approximately 32 ℃. Upper body perfusion was provided by the cardiac output, whereas lower body perfusion was provided by CPB. After clamping the central and peripheral sides of the planned aortic replacement site, descending aortic replacement was performed. After replacement, the clamp was released, and the patient was weaned off CPB. The patient was managed using the same techniques used after total bypass.
We did not use CPB for off-pump CABG. Grafts were harvested before heparin injection. During bypass grafting, hemodynamics were maintained using catecholamine and the loading volume. Norepinephrine was mainly used to stabilize blood pressure. After grafting, protamine was injected to reverse the effect of heparin (1 mg for 200 units of heparin) based on the hypercoagulable status of the patient after off-pump CABG.
Study protocol
After surgery, we attempted to control the systolic blood pressure and CVP to ensure stable conditions during afterload and preload. The target systolic blood pressure, mean CVP, and mean cardiac index were set as 80 to 140 mmHg, 5 to 15 mmHg, and 2.2 L/min/m2, respectively; these values were controlled using vasodilators and catecholamines and by adjusting the infusion volume. The infusion load was adjusted to between 60 and 500 mL/h, depending on the situation. Four catecholamines, dopamine, dobutamine, norepinephrine, and (sometimes) epinephrine, were used almost exclusively; however, when hypotension was not sufficiently controlled, vasopressin and/or phenylephrine were used. Nitroglycerin and nicardipine were used as vasodilators. The heart rate was maintained between 60 and 120 beats per minute using external pacing and beta-blockers. The arterial partial pressure of oxygen was measured hourly, and ventilator settings were adjusted to maintain it at more than 60 mmHg. The carbon dioxide partial pressure was maintained at 30 to 50 mmHg. Patients were not sedated postoperatively; instead, they were extubated and weaned from the ventilator after adequate spontaneous breathing was confirmed on awakening. For cases of pulmonary hypertension with systolic pulmonary artery pressure greater than half the systolic aortic pressure, nitric oxide inhalation therapy was administered; however, these patients were excluded from the study. For cases in which the circulatory status and respiratory status could not be maintained with conservative treatment, intra-aortic balloon pumping and extracorporeal membrane oxygenation were used; however, these patients were excluded from the study as well. If the hemoglobin level decreased to less than 7.0 g/dL, then red blood cell products were administered. Platelet products were administered if the platelet count decreased to less than 50,000/µL. Fresh-frozen plasma was used when postoperative bleeding from the surgical field was more than 100 mL/hour; however, patients with bleeding of more than 300 mL/hour or cardiac tamponade underwent reoperation for hemostasis and were excluded from our study. Vitals were recorded and analyzed for 12 hours postoperatively. We mainly evaluated the differences in the SVRI among the following four types of surgery: MV surgery, AV surgery, TA surgery, and CABG.
Statistical analysis
Each data set was compared between groups using a one-way analysis of variance. Continuous data were compared among the four groups using an analysis of variance every 2 hours postoperatively, and significant differences were compared using the post hoc Tukey-Kramer test. JMP 8.0 software (SAS Institute Inc., Cary, NC, USA) was used, and P<0.05 was considered statistically significant.
Results
A total of 493 patients participated in the study. During this study, 127 underwent MV surgery and 88 underwent simple MV surgery (MV only); 154 underwent AV surgery and 82 underwent simple AV surgery (AV only); 102 underwent TA surgery and 75 underwent simple TA surgery (TA only); and 207 underwent CABG and 145 underwent simple CABG (CABG only). Of the simple MV surgeries, 55 comprised MV plasty and 33 comprised MV replacement. Of the simple AV surgeries, 74 comprised AV replacement for aortic stenosis and 8 comprised AV replacement for aortic regurgitation. Of the simple TA surgeries, 13 comprised ascending aortic replacement, 49 comprised aortic arch replacement, and 3 comprised descending aortic replacement. Thirteen patients underwent simple TA surgery for acute aortic dissection. Of the simple CABG procedures, 127 comprised CABG without CPB and 19 comprised CABG with CPB. The average number of bypasses was 3.21±1.03. The preoperative and intraoperative data of patients who underwent simple cardiac surgeries were compared (Table 1). AV surgery patients were more likely to be female and older than other surgery patients. Although statistically non-significant, the operative time tended to be longer for TA surgery and CABG. The CPB duration was longest for TA surgery, followed by MV surgery and AV surgery. Blood loss was significantly heavier during TA surgery.
Table 1
Surgical type | Patients | Baseline data | ||||
---|---|---|---|---|---|---|
Sex | Age | Operative time (min) | CPB duration (min) | Blood loss (mL) | ||
MV | 88 | Male, 57; female, 31 | 66.82±11.11 | 127.70±227.58 | 301.27±83.54 | 319.14±272.92 |
AV | 82 | Male, 38; female, 44 | 72.60±7.34 | 138.83±213.06 | 252.36±95.47 | 402.75±515.38 |
Off-pump CABG | 127 | Male, 102; female 24 | 67.56±9.28 | 222.21±250.07 | N/A | 361.63±237.43 |
TA | 75 | Male, 53; female, 22 | 68.57±9.84 | 208.94±290.71 | 344.21±102.77 | 688.87±679.35 |
P value (ANOVA) | 0.0003 | 0.0348 | <0.0001 | <0.0001 | ||
P value (Tukey-Kramer) | AV-MV: 0.0005 | TA-AV: <0.0001 | TA-MV: <0.0001 | |||
AV-CABG: 0.0012 | MV-AV: 0.0099 | TA-CABG: <0.0001 | ||||
AV-TA: 0.0411 | TA-MV: 0.0263 | TA-AV: 0.0012 |
Baseline data are reported as the mean ± standard deviation. JMP 8.0 Software (SAS Institute Inc., Cary, NC, USA) was used to perform a one-way ANOVA, followed by the Tukey-Kramer test (P<0.05 was considered statistically significant). CPB, cardiopulmonary bypass; MV, mitral valve surgery; AV, aortic valve surgery; CABG, off-pump coronary artery bypass; N/A, not available; TA, thoracic aorta surgery; ANOVA, analysis of variance.
The SVRI decreased after cardiac surgery and was stabilized within 2 hours of CPB, which may have been attributed to our surgical management (Figure 1). Next, to understand the characteristics of the effects of specific surgeries on SVRI, we compared the SVRI of patients based on the type of surgery they had undergone (Figure 2). SVRI after MV surgery tended to be lower than that after other surgeries, especially after 4 hours postoperatively (P<0.005) (Figure 2A). After AV surgery, the SVRI seemed to be slightly higher than that observed after other procedures (Figure 2B). The SVRI after CABG seemed to change less than that observed with other techniques (Figure 2C). The SVRI immediately after TA surgery was significantly higher (P<0.005); however, with time, it was only slightly higher (Figure 2D).
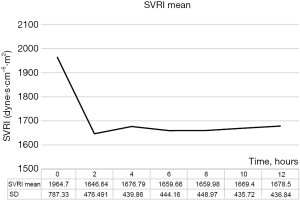
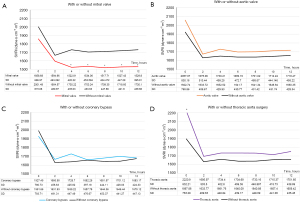
We further analyzed the differences in the SVRI of the four types of surgery (Figure 3, Table 2). Patients who had undergone more than one type of cardiac surgery were excluded from this study (only patients who had undergone simple procedures were included). Of the patients who underwent only CABG, 87.6% underwent off-pump procedures; therefore, only off-pump procedures were selected for comparison. Immediately after surgery, the SVRI tended to be the highest for TA surgery patients, followed by that of patients who underwent AV surgery, MV surgery, and off-pump CABG; however, the difference was not statistically significant. Two hours later, the difference in the mean SVRI almost disappeared because it decreased for all surgery types and remained stable up to 12 hours postoperatively, except in the case of MV surgery. The SVRI for MV surgery alone continued to decrease until 4 hours postoperatively and then stabilized; however, it remained significantly lower than the other postoperative SVRI values. The mean SVRI change at 12 hours postoperatively was the lowest after off-pump CABG, and it remained stable within 200 dyne·s·cm-5·m2; however, a sharp decrease of more than 250 dyne·s·cm-5·m2 was observed after CPB surgery.
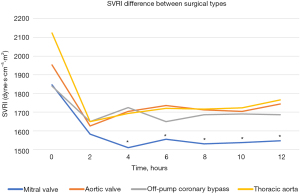
Table 2
Surgical type | Patients | Hours after surgery | ||||||
---|---|---|---|---|---|---|---|---|
0 | 2 | 4 | 6 | 8 | 10 | 12 | ||
MV | 88 | 1,845.69±733.95 | 1,582.08±483.85 | 1,509.92±443.64 | 1,554.69±450.89 | 1,529.88±442.34 | 1,538±476.66 | 1,547.09±417.694 |
AV | 82 | 1,952.13±847.32 | 1,626.43±523.64 | 1,703.98±486.76 | 1,735.5±490.06 | 1,711.55±472.85 | 1,704.01±435.83 | 1,743.78±477.168 |
Off-pump CABG | 127 | 1,839.72±721.48 | 1,649.36±406.58 | 1,723.84±414.06 | 1,648.68±376.08 | 1,685.62±414.8 | 1,689.81±386.07 | 1,686.34±405.09 |
TA | 75 | 2,122.76±804.72 | 1,649.81±486.07 | 1,693±402.30 | 1,719.73±473.51 | 1,715.91±438.42 | 1,723.51±415.60 | 1,764.71±453.51 |
P value (ANOVA) | 0.0586 | 0.7362 | 0.0027 | 0.0322 | 0.0157 | 0.0167 | 0.0055 | |
P value (Tukey-Kramer) | MV-CABG: 0.0025 | MV-AV: 0.0393 | MV-AV: 0.0369 | MV-TA: 0.0297 | MV-TA: 0.0085 | |||
MV-AV: 0.0205 | MV-TA: 0.0369 | MV-AV: 0.0179 | ||||||
MV-TA: 0.0391 |
SVRI data are reported as the mean ± standard deviation. JMP 8.0 software (SAS Institute Inc., Cary, NC, USA) was used to perform the one-way ANOVA, followed by the Tukey-Kramer test (P<0.05 was considered statistically significant). SVRI, systemic vascular resistance index; MV, mitral valve surgery; AV, aortic valve surgery; CABG, off-pump coronary artery bypass; TA, thoracic aorta surgery; ANOVA, analysis of variance.
We also analyzed the differences in systolic blood pressure (Figure 4) and doses of inotropic agents used (dopamine, dobutamine, norepinephrine) among the four surgery types (Figure 5). Blood pressure was especially low immediately after TA surgery (P<0.005). It was also high after AV surgery, especially at 6 to 8 hours postoperatively (P<0.005). Inotrope levels after MV surgery and TA surgery were significantly higher than those after CABG and AV surgery at least 4 hours postoperatively.
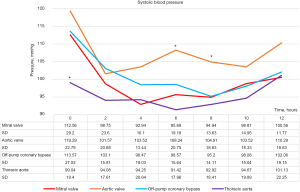
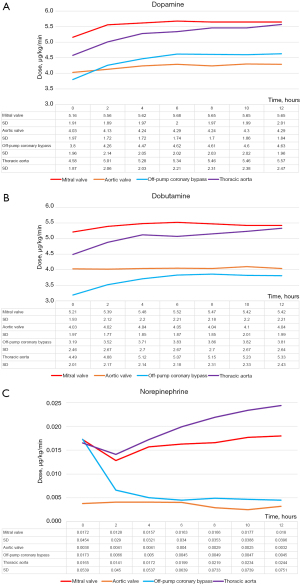
We analyzed the SVRI after on-pump and off-pump CABG (Figure 6). There were no significant differences between them; however, the SVRI after on-pump CABG was relatively higher than that after off-pump CABG.
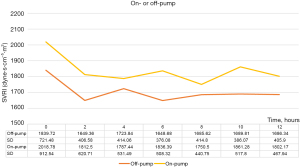
Discussion
To ensure safe postoperative management after cardiac surgery, this study focused on differences in the trends of systemic vascular resistance after cardiac surgery and emphasized the differences observed for each surgical type. Our results indicate differences in postoperative SVRI depending on the type of cardiac surgery performed. First, to consider systemic vascular resistance, it is necessary to consider the impact of CPB, which is essential for many cardiac surgeries. At the start of CPB, the use of clear fluids and an oxygenator causes blood dilution (decrease in blood viscosity and catecholamine concentration) and an inflammatory response (after the release of numerous vasoactive substances), initially decreasing systemic vascular resistance (1-5). However, as surgery continues, the resistance gradually increases, possibly because of increased endogenous catecholamine levels (1,2). At the end of surgery, it is further increased by artificially injected catecholamines to maintain the cardiac function (6). Our results also show that during all cardiac surgeries, the SVRI was initially high (an average of approximately 2,000 dyne·s·cm-5·m2), which may have been attributable to the aforementioned reasons. Thereafter, the SVRI decreased to an average of approximately 1,600 dyne·s·cm-5·m2 and remained stable after 2 hours postoperatively (Figure 1), which may have been attributable to the management of systemic vascular resistance using vasodilators.
Regarding the features of the SVRI curve for each surgery, the curve for MV surgery was distinct. Although we tried to maintain the SVRI at an appropriate level, it decreased persistently. Initially, it was relatively low (approximately 1,800 dyne·s cm-5·m2); then, it decreased further (approximately 1,500 dyne·s·cm-5·m2) and was significantly lower than that observed after the other surgery types (Figures 2A,3) despite the high dose of inotropic agents (Figure 5). For a few cases, we were unable to maintain blood pressure and SVRI using inotropes including vasopressin and phenylephrine. All of these cases involved patients who underwent MV. Therefore, during this study, a sustained decrease in the SVRI occurred after MV. The reason for this phenomenon is unclear; however, it may be related to the vasoplegic syndrome, which involves vasodilatory shock after CPB (3,7-13). Vasoplegic syndrome is characterized by extremely low systemic blood pressure with high cardiac output and low systemic vascular resistance. The risk factors are cardiac surgery for severe heart failure, valve surgery (but not AV surgery), dialysis, advanced age, and the use of diuretics, angiotensin-converting enzyme inhibitors, or heparin. Excessive release of interleukin-8, endothelial cell activation, and vasopressin deficiency are among the possible causes, but the aforementioned risk factors seem to have excessive preload in common. Vasoplegic syndrome stimulates the atrial baroreflex receptor to inhibit the secretion of vasopressin with or without the participation of atrial natriuretic peptide (7,14-18). Therefore, our MV surgery results seem reasonable. MV surgery is characterized by dilating the left atrial wall to secure the visual field during surgery, which may have resulted in a lower SVRI curve after MV surgery. Therefore, the use of vasopressin may be ideal for managing the decreased SVRI after MV surgery. In our next study, we plan to examine the levels of vasopressin and atrial natriuretic peptide after cardiac surgery and to determine the difference between surgical types.
At our hospital, the majority of CABG procedures are performed as off-pump CABG procedures. Therefore, the SVRI results with and without CABG strongly suggest a change in the postoperative SVRI without CPB (Figure 2C). During our study, the change in the SVRI was relatively small after CABG (Figure 3). Blood pressure was also relatively stable without the use of high-dose inotropic drugs (Figures 4,5). This stability may be influenced by the lack of fluctuations in the circulating blood volume and non-physiological effects caused by CPB. Therefore, to observe the influence of CPB, we compared the SVRI of on-pump and that of off-pump CABG; however, we found no significant difference (Figure 6). This may be because of the small number of on-pump CABG (18 cases) and off-pump CABG (127 cases) procedures included in this study.
Considering the SVRI of AV surgery, the trend of its transition is similar to that of other cardiac surgeries and appears to be the result of general postoperative care (Figures 2B,3). However, despite the average SVRI and the use of only relatively small doses of inotropic drugs, blood pressure was relatively high (Figures 4,5). This may be because more than 90% of AV surgeries are performed for aortic stenosis and the patients are released soon after surgery.
The SVRI trend for TA surgery was also similar to that for all surgeries (Figures 2D,3). However, TA surgery was associated with a higher SVRI during the immediate postoperative period compared to other surgeries. This may be because most TA surgeries are performed during cardiac arrest (except for descending aorta replacement) and the CPB duration is long; therefore, the effects of CPB may remain after surgery. The significantly higher blood loss also had an impact. Although significantly higher doses of catecholamines must be used to maintain cardiac function, blood pressure (Figures 4,5), and SVRI, the decrease in the SVRI was not as great as that after MV surgery. This seems to suggest that the decrease in the SVRI after MV surgery is not solely caused by CPB or other surgical interventions.
In summary, SVRI generally declines after cardiac surgery, especially after the use of CPB, but it often stabilizes within a few hours. However, SVRI after MV continues to be lower than that after other procedures. SVRI after off-pump CABG is relatively stable. The decrease in SVRI after AV and TA shows a general post-CPB trend, but blood pressure is higher after AV, while TA requires more catecholamine to maintain blood pressure.
This study had several limitations. First, because it was based on data collected from a single institution, selection bias may have been present. Second, because only historical information was available for this retrospective cohort study, the amount of information available was limited and could not be extended to an analysis of other factors that might cause changes in the SVRI after surgery. However, several studies (1,9) have indicated that MV surgery is a major risk factor for SVRI reduction, thus supporting our hypothesis. We believe that the results of this study are useful for understanding changes in the SVRI and may further improve patient management after cardiac surgery.
Conclusions
There is a significant difference in the SVRI among different types of cardiac surgery. During this study, we highlighted the need for better postoperative care based on the differences in vascular resistance according to the type of cardiac surgery to obtain better postoperative outcomes.
Acknowledgments
Funding: None.
Footnote
Reporting Checklist: The authors have completed the STROBE reporting checklist. Available at https://jtd.amegroups.com/article/view/10.21037/jtd-22-325/rc
Data Sharing Statement: Available at https://jtd.amegroups.com/article/view/10.21037/jtd-22-325/dss
Peer Review File: Available at https://jtd.amegroups.com/article/view/10.21037/jtd-22-325/prf
Conflicts of Interest: Both authors have completed the ICMJE uniform disclosure form (available at https://jtd.amegroups.com/article/view/10.21037/jtd-22-325/coif). The authors have no conflicts of interest to declare.
Ethical Statement:
Open Access Statement: This is an Open Access article distributed in accordance with the Creative Commons Attribution-NonCommercial-NoDerivs 4.0 International License (CC BY-NC-ND 4.0), which permits the non-commercial replication and distribution of the article with the strict proviso that no changes or edits are made and the original work is properly cited (including links to both the formal publication through the relevant DOI and the license). See: https://creativecommons.org/licenses/by-nc-nd/4.0/.
References
- Putnam EA, Manners JM. Vascular resistance during cardiopulmonary bypass. Its effect on cardiac performance in the immediate post-bypass period. Anaesthesia 1983;38:635-43. [Crossref] [PubMed]
- Downing SW, Edmunds LH Jr. Release of vasoactive substances during cardiopulmonary bypass. Ann Thorac Surg 1992;54:1236-43. [Crossref] [PubMed]
- Mekontso-Dessap A, Houël R, Soustelle C, et al. Risk factors for post-cardiopulmonary bypass vasoplegia in patients with preserved left ventricular function. Ann Thorac Surg 2001;71:1428-32. [Crossref] [PubMed]
- Borgdorff P, Fekkes D, Tangelder GJ. Hypotension caused by extracorporeal circulation: serotonin from pump-activated platelets triggers nitric oxide release. Circulation 2002;106:2588-93. [Crossref] [PubMed]
- Miller BE, Levy JH. The inflammatory response to cardiopulmonary bypass. J Cardiothorac Vasc Anesth 1997;11:355-66. [Crossref] [PubMed]
- Ellenberger C, Sologashvili T, Cikirikcioglu M, et al. Risk factors of postcardiotomy ventricular dysfunction in moderate-to-high risk patients undergoing open-heart surgery. Ann Card Anaesth 2017;20:287-96. [Crossref] [PubMed]
- Argenziano M, Chen JM, Choudhri AF, et al. Management of vasodilatory shock after cardiac surgery: identification of predisposing factors and use of a novel pressor agent. J Thorac Cardiovasc Surg 1998;116:973-80. [Crossref] [PubMed]
- Carrel T, Englberger L, Mohacsi P, et al. Low systemic vascular resistance after cardiopulmonary bypass: incidence, etiology, and clinical importance. J Card Surg 2000;15:347-53. [Crossref] [PubMed]
- Tsiouris A, Wilson L, Haddadin AS, et al. Risk assessment and outcomes of vasoplegia after cardiac surgery. Gen Thorac Cardiovasc Surg 2017;65:557-65. [Crossref] [PubMed]
- Patarroyo M, Simbaqueba C, Shrestha K, et al. Pre-operative risk factors and clinical outcomes associated with vasoplegia in recipients of orthotopic heart transplantation in the contemporary era. J Heart Lung Transplant 2012;31:282-7. [Crossref] [PubMed]
- Byrne JG, Leacche M, Paul S, et al. Risk factors and outcomes for 'vasoplegia syndrome' following cardiac transplantation. Eur J Cardiothorac Surg 2004;25:327-32. [Crossref] [PubMed]
- Levin MA, Lin HM, Castillo JG, et al. Early on-cardiopulmonary bypass hypotension and other factors associated with vasoplegic syndrome. Circulation 2009;120:1664-71. [Crossref] [PubMed]
- Kortekaas KA, Lindeman JH, Reinders ME, et al. Pre-existing endothelial cell activation predicts vasoplegia after mitral valve surgery. Interact Cardiovasc Thorac Surg 2013;17:523-30. [Crossref] [PubMed]
- Matsukawa T, Miyamoto T. Angiotensin II-stimulated secretion of arginine vasopressin is inhibited by atrial natriuretic peptide in humans. Am J Physiol Regul Integr Comp Physiol 2011;300:R624-9. [Crossref] [PubMed]
- Greenberg TT, Richmond WH, Stocking RA, et al. Impaired atrial receptor responses in dogs with heart failure due to tricuspid insufficiency and pulmonary artery stenosis. Circ Res 1973;32:424-33. [Crossref] [PubMed]
- Zucker IH, Gorman AJ, Cornish KG, et al. Influence of left ventricular receptor stimulation on plasma vasopressin in conscious dogs. Am J Physiol 1983;245:R792-9. [PubMed]
- Brändle M, Wang W, Zucker IH. Ventricular mechanoreflex and chemoreflex alterations in chronic heart failure. Circ Res 1994;74:262-70. [Crossref] [PubMed]
- Thames MD, Kinugawa T, Smith ML, et al. Abnormalities of baroreflex control in heart failure. J Am Coll Cardiol 1993;22:56A-60A. [Crossref] [PubMed]