Qishen Yiqi dropping pills improve cardiomyocyte hypertrophy via the lncRNA TINCR/miR-193b-3p/RORA axis
Introduction
Owing to the persistent increase in the morbidity and mortality associated with chronic heart failure (CHF), it is currently considered one of the most serious diseases and poses a major threat to affected individuals (1-3). To date, effective drugs for CHF have not been discovered on account of inadequate information on CHF pathogenesis. However, several studies have demonstrated the potential of angiotensin II (Ang II) as an inducer of H9C2 rat cardiomyocyte hypertrophy that promotes apoptosis and reduces cell growth, which can help develop a model of cardiac hypertrophy for laboratory investigations (4,5). Therefore, this model can be used to study the molecular mechanisms underlying CHF in vitro. However, the mechanism of action of lncRNA TINCR in CHF is still unclear.
Traditional Chinese medicine (TCM) is a viable treatment option for CHF due to its low toxicity and physical conditioning potential (6,7). In China, Qishen Yiqi dropping pills (QYDP) [composed of Astragalus membranaceus (Huang qi), Salviae miltiorrhiza (Dan shen), Panax notoginseng (Ren shen), and Lignum dalbergiae odoriferae (Jiang xiang) oil] have been used traditionally for treating cardiovascular diseases (8,9), similar to that for several other TCMs [such as the Qili Qiangxin capsule (10) and Tongsaimai tablet (11)], the mechanism of action and therapeutic effects of QYDP remain unclear.
Reportedly, long non-coding RNAs (lncRNAs) have a close relationship with various cardiovascular diseases, including CHF (12). Findings from several studies have confirmed that the lncRNA terminal differentiation-induced non-coding RNA (TINCR) promotes the development of cancer cells, such as bladder cancer (13) and gastric cancer cells (14). In a study by Li et al., low expression of TINCR was shown to result in cardiomyocyte hypertrophy (15).
Small non-coding RNAs, such as microRNAs (miRNAs), act as powerful mediators in multiple diseases, including cardiovascular diseases (16). The expression of miR-193b-3p, one of the several known miRNAs, can be regulated to control the activity and migration of various types of cancer cells, such as breast cancer (17) and ovarian cancer (18) cells. In addition, Lai et al. (19) demonstrated the therapeutic potential of miR-193b-3p in several diseases of the central nervous system.
The potential of messenger RNAs (mRNAs) in the treatment of genetic diseases and the development of vaccines or gene editing strategies has been demonstrated and is mostly attributed to their safe and effective protein expression profile (20). The RAR-related orphan receptor A (RORA) mRNA was found to exert effects in human developmental disorders (e.g., autism) (21), neurological disorders (such as bipolar disorder) (22), and respiratory diseases (e.g., asthma) (23). The close association between RORA expression and CHF development was demonstrated by Miyazaki et al. (24).
In this study, the interaction among TINCR, miR-193b-3p, and RORA was investigated to explore the potential mechanism by which QYDP protects rat cardiomyocytes from Ang II-induced hypertrophy. We believe our findings provide important theoretical, pharmacological, and molecular biological information necessary for the treatment of CHF. We present the following article in accordance with the MDAR reporting checklist (available at https://jtd.amegroups.com/article/view/10.21037/jtd-22-1322/rc).
Methods
Gene Expression Omnibus (GEO) dataset analysis
Differentially expressed miRNAs were obtained from the GEO sample GSE106547 and divided into two groups: normal group (n=3) and heart failure group (n=3). The differentially expressed mRNAs were obtained from sample GSE16624, which was divided into two groups: normal group (n=3) and heart failure group (n=3). The miRNAs/mRNAs that met the criterion P<0.05 and log2|fold change (FC)| ≥2 were regarded as differentially expressed and used to draw a heatmap. The BiBiServ database (https://bibiserv.cebitec.uni-bielefeld.de/genefisher2/) and TargetScan database (http://www.targetscan.org/vert_72/) were utilized to predict the binding sites of lncRNA/miRNA/mRNA. Raw data (GSE106547 and GSE16624) were download from the GEO website (https://www.ncbi.nlm.nih.gov/geo/).
Cell culture and transfection
After being purchased from the American Type Culture Collection (ATCC, Manassas, VA, USA), H9C2 rat cardiomyocytes were cultivated in Dulbecco’s modified Eagle’s medium (Gibco, Thermo Fisher Scientific, Inc., Waltham, MA, USA), and cultured with 10% fetal bovine serum (FBS) at 37 ℃ and 5% CO2. Once the cells reached 60–80% confluence, a small interfering (si) lncRNA TINCR (si-TINCR) or lncRNA TINCR overexpression plasmid (ov-TINCR) (each 100 nM), miR-193b-3p mimic or inhibitor (each 50 nM), and their negative controls (NCs) were transfected into H9C2 cardiomyocytes by treatment with Lipofectamine® 2000 (Invitrogen, Thermo Fisher Scientific, Inc.) for 4 hours. The H9C2 cells were pretreated with different concentrations of QYDP (0, 10, 50, 100, 250, and 500 µg/mL) as described in our previous study (25), and then stimulated with Ang II (0.1 M; Millipore, Billerica, MA, USA) for 24 hours, as described in a previous study (26). GenePharma Biotechnology Co., Ltd., (Shanghai, China) synthesized the lncRNA TINCR and miR-193b-3p mimic/inhibitor. The primer sequences for TINCR, miR-193b-3p, and RORA were as follows: si-TINCR-1-F, 5'-GGUUGCUAUAAACGUGCAACU-3', si-TINCR-1-R, 5'-UUGCACGUUUAUAGCAACCUG-3'; si-TINCR-2-F, 5'-ACCUUAGCGUGUUCAGAAAUA-3', si-TINCR-2-R, 5'-UUUCUGAACACGCUAAGGUUG-3'; si-TINCR-3-F, 5'-AAGUGUUUCAGCACGUGUAUU-3', si-TINCR-3-R, 5'-UACACGUGCUGAAACACUUUG-3'; si-NC-F, 5'-UUCUCCGAACGUGUCACGUTT-3', si-NC-R, 5'-ACGUGACACGUUCGGAGAATT-3'; miR-193b-3p mimic, 5'-AACUGGCCCACAAAGUCCC-3'; miR-193b-3p mimic NC, 5'-GCUCCCAUCAGCCACAAGA-3'; miR-193b-3p inhibitor, 5'-GGGACUUUGTGGGCCAGUU-3'; miR-193b-3p inhibitor NC, 5'-CAGUACUUUUGUGUAGUACAA-3'.
Hematoxylin-eosin (HE) staining
H9C2 cells were seeded in 6-well plates for cell round coverslip experiment and fixed with 4% paraformaldehyde (Solarbio Science & Technology Co., Ltd.) after 12 hours. After washing twice with PBS, H9C2 cells were stained with hematoxylin (Solarbio Science & Technology Co., Ltd.) at 23±2 ℃, for 4 min, followed by staining with eosin for 90 s, according to the manufacturer's instructions. Then, sections were observed under a light microscope (Olympus Corporation, Tokyo, Japan; magnification, ×200).
Cell Counting Kit-8 (CCK-8) assay
The H9C2 cardiomyocytes were seeded in 96-well plates at 2.5×103 cells/well and cultured for 24–72 hours. An enzyme-labeled instrument (Thermo Fisher Scientific, Inc.) was used to measure the optical density (490 nM) every 24 hours after treatment with the CCK-8 reagent (Solarbio Science & Technology Co., Ltd., Beijing, China), based on the manufacturers’ instructions.
RNA pull-down assay
Lipofectamine® 2000 was used to transfect biotinylated TINCR (Bio-TINCR and Bio-TINCR-Mut), biotinylated miR-193b-3p (Bio-miR-193b-3p and Bio-miR-193b-3p-Mut), and their NCs (Bio-NC or Bio-miR-NC) into H9C2 cells. Then, reverse transcription quantitative polymerase chain reaction (RT-qPCR) was performed for quantitative analysis 10 minutes later when the cell lysate was incubated with Dynabeads M-280 Streptavidin (Invitrogen, Carlsbad, CA, USA) on ice, as described above. Biotinylated RNA was obtained from Sangon Biotech Co., Ltd. (Shanghai, China).
RNA binding protein immunoprecipitation assay
According to the manufacturer’s instructions for the radioimmunoprecipitation (RIP) kit (Millipore), radioimmunoprecipitation assay (RIPA) lysis buffer (Solarbio Science & Technology Co., Ltd.) was added to H9C2 cells for 30 minutes, and 5 µg Argonaute 2 (AGO2) or immunoglobulin G (IgG) antibodies were incubated with magnetic beads for 30 minutes at 20 ℃. Subsequently, it was co-incubated with cell lysate for 4 hours at 4 ℃ and protease K was added at 55 ℃ for 30 minutes before RNA was extracted as described above. Finally, RT-qPCR analysis was performed.
RT-qPCR assays
TRIzol reagent (Invitrogen) was used to extract total RNA from H9C2 cardiomyocytes. The supernatant was discarded after centrifugation at 12,000 ×g (4 ℃, 10 min), and the pellet was suspended in 10 µL of diethyl pyrocarbonate (DEPC) water (Invitrogen), then the PrimeScript RT kit (Takara Bio, Japan) was used to perform reverse transcription. The SYBR® Premix Ex TaqTM II kit (Takara) was used for RT-qPCR analysis with the Applied Biosystems® 7500 Real-Time PCR system (Applied Biosystems, Foster City, CA, USA). The cycling conditions for polymerase chain reaction were as follows: 95 ℃ for 10 minutes, 55 ℃ for 2 minutes, 72 ℃ for 2 minutes, and 40 cycles of 95 ℃ for 15 seconds and 60 ℃ for 32 seconds. The following sequences of the primer pairs were used: TINCR-F, 5'-AAGCTCTCCAGTCAACAGGC-3', TINCR-R, 5'-AAGCTCTCCAGTCAACAGGC-3'; miR-193b-3p-F, 5'-ACACTCCAGCTGGGAACTGGCCCACAA-3', miR-193b-3p-R, 5'-CTCAACTGGTGTCGTGGA-3'; RORA-F, 5'-CATCTCCGCCGATCTCTACA-3', RORA-R, 5'-GTGACTGAGATACCTCGGCTG-3'; U6-F, 5'-CTCGCTTCGGCAGCACA-3', U6-R, 5'-AACGCTTCACGAATTTGCGT-3'; β-actin-F, 5'-CACCCGCGAGTACAACCTTC-3', β-actin-R, 5'-CCCATACCCACCATCACACC-3'. By using the 2−ΔΔCt method (27), the RNA levels were normalized to those of β-actin or U6 and calculated.
Western blotting
According to the manufacturer’s instructions, we used a lysis buffer (Solarbio Science & Technology Co., Ltd.) to lyse H9C2 cells, and measured the protein concentration by the bicinchoninic acid (BCA) protein assay kit (Solarbio Science & Technology Co., Ltd.). To separate the proteins (20 µg), sodium dodecyl sulfate-polyacrylamide gel electrophoresis (SDS-PAGE; 10%) was performed, and then the proteins were transferred to polyvinylidene fluoride (PVDF) membranes (EMD Millipore), which were rinsed with Tris buffered saline + 0.05% Tween 20 (Solarbio Science & Technology Co., Ltd.), blocked with 5% bovine serum albumin (BSA; Solarbio Science & Technology Co., Ltd.), and treated overnight (4 ℃) with an anti-RORA (1:1,000; ab267787; Abcam, Cambridge, AM, USA) antibody (used as the primary antibody). Then, the membranes were taken as a secondary antibody (goat anti-rabbit; 1:1,000; ab205718; Abcam) for 2 hours at 23±2 ℃, and an anti-GAPDH antibody (1:10,000; cat. No. ab181602; Abcam) was used as the loading control.
Luciferase reporter assay
As TargetScan and BiBiServ databases’ combined prediction results, miR-193b-3p can bind to TINCR and RORA. For constructing the recombinant luciferase reporter vector, the sequences of the RORA and TINCR were inserted into the pmirGLO vector (Promega Corp., Madison, WI, USA). To construct the mutant (mut) TINCR and mut RORA plasmids, the core sequence of the miR-193b-3p-binding sites were mutated. Then, the wild-type (WT) and mut pmirGLO plasmids were co-transfected into H9C2 cells with the miR-193b-3p mimic NC or miR-193b-3p mimic by Lipofectamine® 2000. An enzyme-labeled instrument was used to measure luciferase activity, and we used the ratio of firefly luciferase activity to Renilla luciferase activity to normalize the values of firefly luciferase activity.
Statistical analysis
Data were presented as means ± standard deviations from triplicates. Based on one-way analysis of variance (ANOVA) and Bonferroni’s post-hoc test, statistical analysis was performed. A Student’s t-test was used for independent two-group analyses. Differences were considered significant at P<0.05.
Results
QYDP regulates the proliferation and surface area of H9C2 cardiomyocytes through TINCR
As observed in the CCK-8 assay, the proliferation of H9C2 cardiomyocytes decreased in response to treatment with 250 and 500 µg/mL QYDP (Figure 1A), but not with QYDP administered at lower concentrations (0, 10, 50, and 100 µg/mL), suggesting that at a concentration equal to or more than 250 µg/mL (for 4 h), QYDP hindered the proliferation of H9C2 cardiomyocytes. To prevent interference in cell proliferation at excess QYDP concentrations, we selected 100 µg/mL of QYDP administered for 4 hours as the treatment condition for the subsequent experiments. Next, H9C2 cardiomyocytes were treated with Ang II to develop the cardiac hypertrophy model. After Ang II treatment, the expression of TINCR in H9C2 cardiomyocytes was significantly reduced (Figure 1B), and the surface area increased (Figure 1C). After QYDP (0, 10, 50, and 100 µg/mL) treatment, the expression of TINCR was up-regulated and the surface area of the cells decreased. These results suggest that TINCR may be involved in the process of cardiomyocyte hypertrophy.
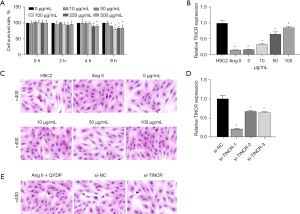
TINCR inhibition reverses the effects of QYDP
To further explore the association between TINCR expression and QYDP, we constructed three si-TINCR sequences to inhibit TINCR expression and transfected H9C2 cardiomyocytes with these. According to the result of RT-qPCR experiments, the expression of TINCR was downregulated in the si-TINCR transfection group (Figure 1D), indicating the successful synthesis of si-TINCR. Of note, si-TINCR-1 exhibited the greatest inhibition efficiency; hence, it was the TINCR inhibitor used in the subsequent experiments. The surface area of H9C2 cardiomyocytes increased significantly in the si-TINCR transfection group (Figure 1E). The findings suggested that QYDP improved Ang II-induced H9C2 cardiomyocyte hypertrophy, and TINCR inhibition reversed the effects of QYDP.
TINCR is bound to miR-193b-3p
Through GEO data analysis of the sample (GSE106547), it was found that 25 miRNAs were differentially expressed (Figure 2A). Subsequently, upon analyzing the miRNAs bound to TINCR using the BiBiServ database, it showed that miR-193b-3p is closely associated with TINCR (Figure 2B). The subsequent RT-qPCR results showed that the expression of miR-193b-3p increased after Ang II induction, and gradually decreased under the action of QYDP (Figure 2C). Additionally, the dual luciferase analysis showed that following the co-transfection of miR-193b-3p and TINCR, the fluorescence intensity of the cells in the WT TINCR + mimic group decreased significantly compared to that of the cells in the WT TINCR + mimic NC group (P<0.05). However, there was no significant change in the fluorescence intensity between the Mut TINCR + mimic NC group and the Mut TINCR + mimic group (P>0.05) (Figure 2D). The RNA pull-down assay showed that TINCR and miR-193b-3p expression in H9C2 cardiomyocytes was evidently higher in the WT groups than in the control and MUT groups (Figure 2E,2F). In addition, RT-qPCR analysis revealed that si-TINCR transfection promoted miR-193b-3p expression, but the miR-193b-3p inhibitor exerted no significant effect on TINCR expression (Figure 2G,2H). We speculated that TINCR adsorbed miR-193b-3p by acting as a competitive endogenous RNA (ceRNA) sponge. However, the effects on the downstream targets remained unclear.
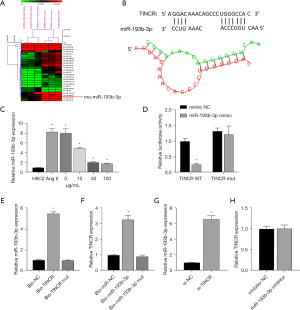
miR-193b-3p directly targets RORA
Through the GEO data analysis of the sample (GSE16624), it was found that there were 337 differentially expressed mRNAs (Figure 3A). The TargetScan database revealed that there were 190 hypothetical target genes downstream of miR-193b-3p. The collection of the two databases has only one gene, which is RORA (Figure 3B); we chose RORA to do further research on molecular mechanism. As observed in the double luciferase assay, the fluorescence intensity in the WT RORA + mimic NC group was evidently lower than that in the WT RORA + mimic group (P<0.05). The fluorescence intensity in the MUT RORA+ mimic group showed no significant difference (P>0.05) with that in the MUT RORA+ mimic NC group (Figure 3C). The RIP results showed that in H9C2 cells, the enrichment degree of miR-140-3p and PIK3CA in AGO2 group was higher than that of IgG in control group (Figure 3D). These results demonstrated that miR-193b-3p directly targets RORA.
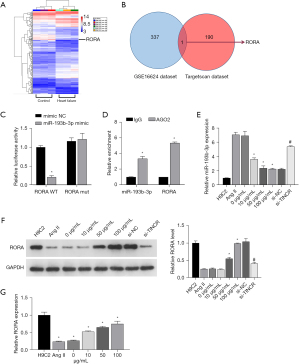
QYDP improves Ang II-induced damage through the TINCR/miR-193b-3p axis
To further investigate the mechanism by which TINCR/miR-193b-3p enhances the effects of QYDP in Ang II-induced hypertrophy, we treated the Ang II-induced H9C2 cardiomyocytes with QYDP and si-TINCR simultaneously. Based on the RT-qPCR and western blotting experiments’ results, Ang II induced and promoted the expression of miR-193b-3p (Figure 3E) and reduced the expression of RORA protein (Figure 3F). Treatment with QYDP reversed the effects induced by Ang II, whereas the inhibition of TINCR negated the effects of QYDP. In addition, Ang II induction also led to a decrease in the expression of RORA, and QYDP treatment promoted the recovery of RORA levels (Figure 3G).
QYDP regulates the TINCR/miR-193b-3p/RORA axis
For further investigation of the mechanism of action of TINCR and miR-193b-3p, we constructed the TINCR overexpression plasmid ov-TINCR and transfected H9C2 cardiomyocytes with it. The expression of TINCR increased in the ov-TINCR transfection group (Figure 4A). This indicated the successful construction of ov-TINCR. To confirm the interaction among TINCR, miR-193b-3p, and RORA, we co-transfected a RORA overexpression plasmid with an miR-193b-3p mimic. Ang II induced the downregulation of TINCR (Figure 4B), upregulation of miR-193b-3p (Figure 4C), the decrease of RORA protein levels (Figure 4D), and expansion of the surface area of H9C2 cardiomyocytes (Figure 4E), but ov-TINCR reversed the effects of Ang II induction. However, the miR-193b-3p mimic negated the effects of ov-TINCR. Above all, the interconnectedness in the regulation of TINCR, miR-193b-3p, and RORA was indicated.
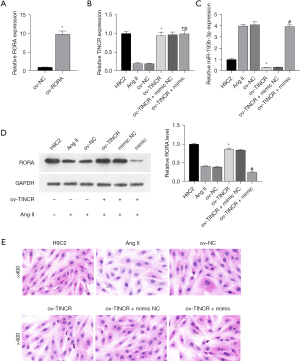
In conclusion, QYDP improved H9C2 cardiomyocyte hypertrophy and increase cell proliferation rate through the TINCR/miR-193b-3p/RORA axis.
Discussion
An increasing number of patients face the risk of CHF development, and healthcare systems worldwide are aiming for a better understanding of CHF pathology and its treatment (28-30). The therapeutic effects of TCM or TCM extracts in cardiovascular diseases (e.g., coronary heart disease and hypertension) (31) have been investigated in several studies, and TCM or TCM extracts have been shown to stabilize the physiological conditions of patients and improve cardiac function (32). In this study, we investigated the function and potential mechanism of action of a TCM in a CHF model. Among different TCMs, Dalbergia otoginsen was shown to suppress angina pectoris (33), Salvia otoginsenge was shown to exhibit nerve-protecting effects (34), and Panax otoginseng was shown to suppress apoptosis (10). These three TCMs are also the primary components of QYDP. Therefore, QYDP could be an effective drug for CHF. In addition, this study also showed that QYDP alleviated the downregulation of TINCR expression and H9C2 cardiomyocyte hypertrophy induced by Ang II. This result is similar to that reported by Qiu et al. (35). Our results showed that QYDP improved Ang II-induced H9C2 cardiomyocyte damage, which was reversed upon the downregulation of TINCR, which is similar to those reported by Zhang et al. (36). The findings of this study suggested that QYDP improved H9C2 cardiomyocyte hypertrophy by upregulating TINCR expression.
To further understand the role of TINCR in the protective effect of QYDP on H9C2 cardiomyocytes, we explored the regulation of downstream miRNAs and mRNAs by TINCR. Our findings confirmed for the first time that TINCR acts as a ceRNA sponge by adsorbing miR-193b-3p. Subsequently, miR-193b-3p was found to directly target RORA and regulate its transcription and translation. It was revealed that QYDP upregulated TINCR expression and improved cardiomyocyte hypertrophy, possibly through the TINCR/miR-193b-3p/RORA axis. In subsequent cell experiments, the low expression of TINCR increased the miR-193b-3p levels, decreased RORA expression, promoted H9C2 cardiomyocyte hypertrophy, and reversed the protective effects of QYDP, which further confirmed our hypothesis. Additionally, the miR-193b-3p mimic reversed the effects of TINCR overexpression. The RIP experiment also confirmed that AGO2 enriched miR-193b-3p and RORA to a much higher degree than IgG. Based on these findings, we confirmed that QYDP improves H9C2 cardiomyocyte hypertrophy through the TINCR/miR-193b-3p/RORA axis.
This study had certain limitations. First, the effect of QYDP on Ang II-induced cardiomyocyte hypertrophy was not analyzed in an animal model. Second, we did not conduct a thorough analysis of the downstream signal transduction affected by the TINCR/miR-193b-3p/RORA axis.
In conclusion, QYDP was shown to protect H9C2 cardiomyocytes from Ang II-induced hypertrophy through the TINCR/miR-193b-3p/RORA axis. Our findings confirmed the effectiveness of QYDP in the treatment of CHF and may serve as the pharmacological and molecular biological basis for the treatment of CHF using TCM.
Acknowledgments
Funding: This work was supported by Key Research and Development of Hainan Province (Social development direction) (No. ZDYF2019121).
Footnote
Reporting Checklist: The authors have completed the MDAR reporting checklist. Available at https://jtd.amegroups.com/article/view/10.21037/jtd-22-1322/rc
Data Sharing Statement: Available at https://jtd.amegroups.com/article/view/10.21037/jtd-22-1322/dss
Conflicts of Interest: All authors have completed the ICMJE uniform disclosure form (available at https://jtd.amegroups.com/article/view/10.21037/jtd-22-1322/coif). The authors have no conflicts of interest to declare.
Ethical Statement: The authors are accountable for all aspects of the work in ensuring that questions related to the accuracy or integrity of any part of the work are appropriately investigated and resolved.
Open Access Statement: This is an Open Access article distributed in accordance with the Creative Commons Attribution-NonCommercial-NoDerivs 4.0 International License (CC BY-NC-ND 4.0), which permits the non-commercial replication and distribution of the article with the strict proviso that no changes or edits are made and the original work is properly cited (including links to both the formal publication through the relevant DOI and the license). See: https://creativecommons.org/licenses/by-nc-nd/4.0/.
References
- Ewen S, Nikolovska A, Zivanovic I, et al. Chronic heart failure - new insights. Dtsch Med Wochenschr 2016;141:1560-4. [PubMed]
- Špinar J, Špinarová L, Vítovec J. Pathophysiology, causes and epidemiology of chronic heart failure. Vnitr Lek Fall;64:834-8.
- Authors/Task Force Members. 2021 ESC Guidelines for the diagnosis and treatment of acute and chronic heart failure: Developed by the Task Force for the diagnosis and treatment of acute and chronic heart failure of the European Society of Cardiology (ESC). With the special contribution of the Heart Failure Association (HFA) of the ESC. Eur J Heart Fail 2022;24:4-131. [Crossref] [PubMed]
- Ye S, Luo W, Khan ZA, et al. Celastrol Attenuates Angiotensin II-Induced Cardiac Remodeling by Targeting STAT3. Circ Res 2020;126:1007-23. [Crossref] [PubMed]
- Li S, Zhu Z, Xue M, et al. Fibroblast growth factor 21 protects the heart from angiotensin II-induced cardiac hypertrophy and dysfunction via SIRT1. Biochim Biophys Acta Mol Basis Dis 2019;1865:1241-52. [Crossref] [PubMed]
- Oravecz M, Mészáros J. Traditional Chinese medicine: theoretical background and its use in China. Orv Hetil 2012;153:723-31. [Crossref] [PubMed]
- Shao-Mei W, Li-Fang Y, Li-Hong W. Traditional Chinese medicine enhances myocardial metabolism during heart failure. Biomed Pharmacother 2022;146:112538. [Crossref] [PubMed]
- Lu Y, Wang D, Yuan X, et al. Protective effect of Qishen Yiqi dropping pills on the myocardium of rats with chronic heart failure. Exp Ther Med 2019;17:378-82. [PubMed]
- Sun J, Qian H, Li X, et al. QishenYiqi Dripping Pill Improves Heart Failure by Up-Regulation of β2-Adrenergic Receptor Expression. J Heart Valve Dis 2017;26:193-9. [PubMed]
- Wang Y, Wang Q, Li C, et al. A Review of Chinese Herbal Medicine for the Treatment of Chronic Heart Failure. Curr Pharm Des 2017;23:5115-24. [PubMed]
- Cao YM, Wang YZ, Pei XH, et al. Expert consensus on Tongsaimai Tablets/Capsules in treatment of peripheral vascular diseases in clinical practice. Zhongguo Zhong Yao Za Zhi 2021;46:6568-73. [PubMed]
- Zhang X, Gao Y, Wu H, et al. LncRNA HOX transcript antisense RNA mitigates cardiac function injury in chronic heart failure via regulating microRNA-30a-5p to target KDM3A. J Cell Mol Med 2022;26:1473-85. [Crossref] [PubMed]
- Xu C, Liu M, Jia D, et al. lncRNA TINCR SNPs and Expression Levels Are Associated with Bladder Cancer Susceptibility. Genet Test Mol Biomarkers 2021;25:31-41. [Crossref] [PubMed]
- Ma X, Huang C, Luo D, et al. Tag SNPs of long non-coding RNA TINCR affect the genetic susceptibility to gastric cancer in a Chinese population. Oncotarget 2016;7:87114-23. [Crossref] [PubMed]
- Li H, Shi H, Zhang F, et al. LncRNA Tincr regulates PKCɛ expression in a miR-31-5p-dependent manner in cardiomyocyte hypertrophy. Naunyn Schmiedebergs Arch Pharmacol 2020;393:2495-506. [Crossref] [PubMed]
- Chistiakov DA, Sobenin IA, Orekhov AN. Strategies to deliver microRNAs as potential therapeutics in the treatment of cardiovascular pathology. Drug Deliv 2012;19:392-405. [Crossref] [PubMed]
- Hafstað V, Søkilde R, Häkkinen J, et al. Regulatory networks and 5' partner usage of miRNA host gene fusions in breast cancer. Int J Cancer 2022;151:95-106. [Crossref] [PubMed]
- Hamidi F, Gilani N, Belaghi RA, et al. Exploration of Potential miRNA Biomarkers and Prediction for Ovarian Cancer Using Artificial Intelligence. Front Genet 2021;12:724785. [Crossref] [PubMed]
- Lai N, Wu D, Liang T, et al. Systemic exosomal miR-193b-3p delivery attenuates neuroinflammation in early brain injury after subarachnoid hemorrhage in mice. J Neuroinflammation 2020;17:74. [Crossref] [PubMed]
- Guan S, Rosenecker J. Nanotechnologies in delivery of mRNA therapeutics using nonviral vector-based delivery systems. Gene Ther 2017;24:133-43. [Crossref] [PubMed]
- Hu VW, Sarachana T, Sherrard RM, et al. Investigation of sex differences in the expression of RORA and its transcriptional targets in the brain as a potential contributor to the sex bias in autism. Mol Autism 2015;6:7. [Crossref] [PubMed]
- Lai YC, Kao CF, Lu ML, et al. Investigation of associations between NR1D1, RORA and RORB genes and bipolar disorder. PLoS One 2015;10:e0121245. [Crossref] [PubMed]
- Cai X, Lin M, Cao S, et al. The association of RAR-related orphan receptor A (RORA) gene polymorphisms with the risk of asthma. Ann Hum Genet 2018;82:158-64. [Crossref] [PubMed]
- Miyazaki M, Schroder E, Edelmann SE, et al. Age-associated disruption of molecular clock expression in skeletal muscle of the spontaneously hypertensive rat. PLoS One 2011;6:e27168. [Crossref] [PubMed]
- Luo Y, Chen J, Chen Y, et al. Qishen Yiqi dropping pills improve isoproterenol-induced cardiomyocyte hypertrophy by regulating X-inactive specific transcript (XIST) expression in rats. J Thorac Dis 2022;14:2213-23. [Crossref] [PubMed]
- Zhang L, Deng M, Lu A, et al. Sodium butyrate attenuates angiotensin II-induced cardiac hypertrophy by inhibiting COX2/PGE2 pathway via a HDAC5/HDAC6-dependent mechanism. J Cell Mol Med 2019;23:8139-50. [Crossref] [PubMed]
- Livak KJ, Schmittgen TD. Analysis of relative gene expression data using real-time quantitative PCR and the 2(-Delta Delta C(T)) Method. Methods 2001;25:402-8. [Crossref] [PubMed]
- Mascolo A, di Mauro G, Cappetta D, et al. Current and future therapeutic perspective in chronic heart failure. Pharmacol Res 2022;175:106035. [Crossref] [PubMed]
- Bauersachs J, Soltani S. Guidelines of the ESC 2021 on heart failure. Herz 2022;47:12-8. [Crossref] [PubMed]
- Triposkiadis F, Xanthopoulos A, Parissis J, et al. Pathogenesis of chronic heart failure: cardiovascular aging, risk factors, comorbidities, and disease modifiers. Heart Fail Rev 2022;27:337-44. [Crossref] [PubMed]
- Wu GS, Li HK, Zhang WD. Metabolomics and its application in the treatment of coronary heart disease with traditional Chinese medicine. Chin J Nat Med 2019;17:321-30. [Crossref] [PubMed]
- Jia Q, Wang L, Zhang X, et al. Prevention and treatment of chronic heart failure through traditional Chinese medicine: Role of the gut microbiota. Pharmacol Res 2020;151:104552. [Crossref] [PubMed]
- Zhao X, Wang C, Meng H, et al. Dalbergia odorifera: A review of its traditional uses, phytochemistry, pharmacology, and quality control. J Ethnopharmacol 2020;248:112328. [Crossref] [PubMed]
- Su CY, Ming QL, Rahman K, et al. Salvia miltiorrhiza: Traditional medicinal uses, chemistry, and pharmacology. Chin J Nat Med 2015;13:163-82. [Crossref] [PubMed]
- Qiu Q, Lin Y, Xiao C, et al. Time-Course of the Effects of QSYQ in Promoting Heart Function in Ameroid Constrictor-Induced Myocardial Ischemia Pigs. Evid Based Complement Alternat Med 2014;2014:571076. [Crossref] [PubMed]
- Zhang Y, Yu J, Zhang W, et al. An integrated evidence-based targeting strategy for determining combinatorial bioactive ingredients of a compound herbal medicine Qishen Yiqi dripping pills. J Ethnopharmacol 2018;219:288-98. [Crossref] [PubMed]
(English Language Editor: J. Jones)