Recipient, donor, and surgical factors leading to primary graft dysfunction after lung transplant
Highlight box
Key findings
• Preoperative and intraoperative risk factors of primary graft dysfunction (PGD) grade 1 and 2 were similar to those of PGD grade 3.
What is known and what is new?
• Various risk factors of PGD grade 3 have been reported, but it is unknown if risk factors of PGD grade 1 and 2 are differences from its PGD grade 3.
• Acute respiratory distress syndrome, operative time, and intraoperative veno-arterial extracorporeal membrane oxygenator use were common risk factors for PGD grade 1 or 2 and grade 3.
What is the implication, and what should change now?
• PGD grade 1 or 2 and PGD grade 3 are certainly a constant disease group with common risk factors.
Introduction
Primary graft dysfunction (PGD) is a major cause of early mortality following lung transplantation (LT). PGD is defined as the syndrome of acute lung injury early after LT (1). The International Society for Heart and Lung Transplantation (ISHLT) working group on PGD proposed a consensus-based standardized definition and grading system. This definition has since been used to characterize PGD clinically by diffuse alveolar infiltrates on chest X-ray (CXR) imaging. The degree of associated hypoxemia determines its severity which is graded on a scale of 0 to 3 (1). In 2016, the ISHLT working group updated guidelines which clarified the grading and added extubated patients by advocating for using the patient’s estimated FiO2 in the PaO2/FiO2 ratio rather than CXR findings alone, regardless of the mode of non-invasive ventilation (2). Additional clarifications included the use of a saturation scale for patients who may not have an arterial monitoring line, the grading of patients on extracorporeal membrane oxygenation (ECMO), and improved clarity of CXR findings (2). In addition to early mortality, PGD could lead to late mortality including chronic lung allograft dysfunction (3,4).
Majority reports have assessed the risk factors for PGD without distinguishing between the severity of grade for PGD after LT. In this study, we sought to identify recipient, donor, and surgical risk factors specifically associated with mild/moderate or severe PGD. We also analyzed the incidence of morbidity and postoperative complications based on the severity of PGD, including intensive care unit (ICU) stays, hospital stays, stroke, acute kidney injury (AKI), as well as overall mortality. We present the following article in accordance with the STROBE reporting checklist (available at https://jtd.amegroups.com/article/view/10.21037/jtd-22-974/rc).
Methods
Study design
Patient data was collected retrospectively using the electronic medical record and stored in a database at Northwestern University Medical Center in Chicago, Illinois. Adult patients undergoing lung transplantation at our institution between March 2018 and September 2021 were included. Multi-organ transplant recipients were excluded from this study. All bilateral lung transplants were performed via clamshell incisions, and all single lung transplantations were performed via anterior thoracotomies. All patients received regular follow-up in our outpatient clinic after discharge. Survival after lung transplantation was documented using both in-person and telemedicine throughout the study period. Data on clinical characteristics and laboratory results, as well as treatment and outcomes, were obtained by the study team from electronic medical records and reviewed by trained physicians. Information recorded included demographic data, medical history, underlying comorbidities, laboratory findings, medical course, and treatments administered. Intraoperative procedures and postoperative complications were also recorded. Patient demographics, postoperative complications, and outcomes were compared among the PGD groups; and they were divided into three categories: those who had PGD grade 0; those who had PGD grade 1 or 2; and those who had PGD grade 3 after LT. This study was approved by the Northwestern University Institutional Review Board (No. STU00207250). The need for patient consent for data collection was waived by the Institutional Review Board as this was a retrospective study. The study was conducted in accordance with the Declaration of Helsinki (as revised in 2013).
Definitions of complications
Complications following lung transplant were defined using the following criteria:
Primary graft dysfunction
Patients with no evidence of pulmonary edema on CXR are considered grade 0. Absence of invasive mechanical ventilation was graded according to the PaO2/FiO2 ratio, using methods similar to those receiving mechanical ventilation. If PaO2 was not available for calculation of a PaO2/FiO2 ratio, then an oxygen saturation/FiO2 ratio was used to calculate. Grade 1; PaO2/FiO2 ratio is >300, Grade 2; PaO2/FiO2 ratio is 200–300, Grade 3; PaO2/FiO2 ratio is <200. The worst PaO2/FiO2 ratio within 72 hours after LT was used. Use of ECMO with bilateral pulmonary edema on CXR image was graded as grade 3. The continuous use of ECMO without pulmonary edema on CXR imaging was excluded (2).
AKI
AKI definition used the Risk, Failure, Loss of kidney function, and End-stage kidney disease classification (5).
Stroke
Stroke was defined as a new deficit confirmed via abnormal neuroimaging, (either computed tomography scan or magnetic resonance imaging), which was confirmed by neurologist. This was further divided into ischemic or hemorrhagic causes.
Ischemic complication
Ischemic complication was defined as a new diagnosis of thrombotic events including bowel ischemia and limb ischemia.
Preoperative veno-venous(VV)-ECMO management
Patients did not receive continuous anticoagulation unless there was a specific indication, such as deep venous thrombosis or pulmonary embolism, and as in our previous study (6), bleeding parameters such as activated clotting time and activated partial thromboplastin time were not monitored. Patients not receiving continuous systemic anticoagulation received unfractionated heparin 5,000 U subcutaneously every 8 hours to prevent deep vein thrombosis. VV-ECMO flow was maintained at a minimum of 3.0–3.5 L/min, consistent with our recent reports, to reduce thrombotic complications in the ECMO circuit (6-8). Different cannulation strategies [Internal jugular vein-femoral vein cannulation vs. ProtekDuo® cannulation (CardiacAssist Inc., Pittsburgh, PA, USA)] were used in patients according to surgeon preference. The VV-ECMO circuit included a Quadrox-iD adult (7.0) oxygenator (MAQUET Holding B.V. & Co. KG, Germany) and Rotaflow pump (MAQUET Holding B.V. & Co. KG, Germany). All components of the ECMO circuit were heparin-coated except for the cannulas.
Statistical analysis
The sample size was equal to the number of patients treated during the study period and no statistical sample size was calculated. There were no missing data in the institutional cohorts. Continuous variables were compared using t-test and one-way analysis of variance test while categorical variables were compared using chi-square test. P values <0.05 were accepted as statistically significant. Logistic regression analysis was performed to determine odds ratios (OR) and 95% confidence intervals (CI). To build our models, we performed a univariate analysis and included all predictors if the test had a P value of 0.10 or less. In the etiology category, only one variable was picked up for multivariate analysis. The Kaplan-Meier method was used to estimate survival, and a Wilcoxon test was performed to compare survival between the groups. As a sensitivity analysis, a univariate and a multivariate analysis were performed between all PGD grades and the PGD grade 0 group. Receiver operating characteristic (ROC) curves were used to calculate cutoff points for risk of PGD and area under the curve (AUC). Statistical analysis was performed using EZR (Saitama Medical Center, Jichi Medical University, Japan), a GUI in R (The R Foundation for Statistical Computing, Vienna, Austria) (9).
Results
Study population and clinical characteristics of lung transplant recipient
A total of 151 consecutive patients were included in the study, with no excluded cases. All donor lungs were donated after brain death and no ex vivo lung perfusions were performed. All recipients had no prior history of cardiac surgery or LT. Eighty three of 151 patients (55.0%) were evaluated as PGD grade 0 groups. Similarly, 45 patients (29.8%) had grade 1 or 2, and 23 patients (15.2%) had PGD grade 3 after LT. Of the 23 patients with PGD grade 3, 21 of them were supported with ECMO after LT, and 2 patients were graded PGD grade 3 based on the low PaO2/FiO2 ratio without ECMO. The mean follow-up period for all patients was 686.2±367.1 days. PGD grade 3 patients were younger than grade 0 patients (59.0±11.0 years old in grade 0 vs. 51.7±13.9 years in grade 3, P=0.02). Gender and smoking history were not significantly different among the three groups. More VV-ECMO was used before LT in PGD grade 3 than in the other groups, and more bilateral LT were performed in PGD grades 1 to 3 (grade 0 vs. 1 or 2 vs. 3, pre VV-ECMO use, 6.0% vs. 11.1% vs. 52.2%, P<0.001; bilateral, 54.2% vs. 84.4% vs. 73.9%, P<0.01; respectively). Coronavirus disease 2019 (COVID-19) associated acute respiratory distress syndrome (ARDS) was a more common indication for LT in PGD grades 1 to 3 than PGD grade 0 (grade 0 vs. 1 or 2 vs. 3, 10.8% vs. 28.9% vs. 34.8%, P<0.01). In the laboratory data, PGD grade 3 patients had lower hemoglobin and higher total bilirubin than the other groups (P=0.04, P<0.001, respectively). Furthermore, PaO2 was lower in the PGD grade 3 patients than PGD grade 0 patients (294.6±111.4 mmHg in grade 0 vs. 214.9±123.0 in grade 3, P=0.02). There was no significant different in the donor age, gender, or cause of death among the three groups (Table 1).
Table 1
Variable | PGD grade 0 (n=83) | PGD grade 1/2 (n=45) | PGD grade 3 (n=23) | P value |
---|---|---|---|---|
Recipient factors | ||||
Age, years | 59.0±11.0 | 54.7±13.9 | 51.7±13.9 | 0.02 |
Female | 35 (42.2%) | 18 (40.0%) | 14 (60.9%) | 0.22 |
BMI, kg/m2 | 25.3±4.5 | 25.9±5.0 | 26.3±4.6 | 0.61 |
BSA, m2 | 1.8±0.2 | 1.8±0.2 | 1.9±0.2 | 0.93 |
Smoking history | 35 (42.2%) | 15 (33.3%) | 7 (30.4%) | 0.45 |
Hypertension | 44 (53.0%) | 21 (46.7%) | 10 (43.5%) | 0.64 |
Diabetes | 30 (36.1%) | 11 (24.4%) | 8 (34.8%) | 0.39 |
CKD | 8 (9.6%) | 2 (4.4%) | 4 (17.4%) | 0.22 |
Pre VV-ECMO use | 5 (6.0%) | 5 (11.1%) | 12 (52.2%) | <0.001 |
Bilateral | 45 (54.2%) | 38 (84.4%) | 17 (73.9%) | <0.01 |
Etiology | ||||
ILD | 33 (39.8%) | 10 (22.2%) | 10 (43.5%) | 0.09 |
COPD/Emphysema | 23 (27.7%) | 7 (15.6%) | 1 (4.3%) | 0.03 |
ARDS (COVID-19) | 9 (10.8%) | 13 (28.9%) | 8 (34.8%) | <0.01 |
PAH | 8 (9.6%) | 12 (26.7%) | 2 (8.7%) | 0.02 |
Bronchitis/Bronchiectasis | 4 (4.8%) | 3 (6.7%) | 1 (4.3%) | 0.88 |
CF | 6 (7.2%) | 0 (0%) | 1 (4.3%) | 0.18 |
Laboratory | ||||
Hemoglobin, g/dL | 11.2±2.6 | 11.3±2.5 | 9.8±2.6 | 0.04 |
WBC, 1,000/mm3 | 10.8±4.4 | 9.4±3.3 | 10.5±4.1 | 0.17 |
Platelets, 1,000/mm3 | 255.8±113.6 | 245.8±98.5 | 196.8±95.3 | 0.07 |
Sodium, mEq/L | 139.5±3.1 | 140.1±3.4 | 140.9±4.1 | 0.21 |
BUN, mg/dL | 16.0±6.8 | 16.2±9.0 | 20.4±11.7 | 0.07 |
Creatinine, mg/dL | 0.74±0.19 | 0.72±0.20 | 0.79±0.28 | 0.46 |
ALT, U/L | 21.7±24.7 | 19.3±10.5 | 25.4±17.6 | 0.52 |
AST, U/L | 31.9±27.4 | 25.2±10.1 | 37.5±31.3 | 0.14 |
Albumin, g/dL | 4.4±4.7 | 5.4±9.8 | 3.4±0.8 | 0.46 |
Total bilirubin, mg/dL | 0.7±0.5 | 0.6±0.3 | 1.2±1.3 | <0.01 |
INR | 1.1±0.2 | 1.1±0.2 | 1.2±0.5 | 0.22 |
Arterial blood gas | ||||
pH | 7.37±0.07 | 7.37±0.07 | 7.38±0.09 | 0.94 |
PaCO2 | 51.3±11.6 | 49.8±13.8 | 47.2±13.2 | 0.36 |
PaO2 | 294.6±111.4 | 264.5±128.1 | 214.9±123.0 | 0.02 |
Donor | ||||
Age, years | 32.0±12.0 | 33.0±11.4 | 31.7±11.7 | 0.88 |
Female | 31 (37.8%) | 10 (22.7%) | 10 (45.5%) | 0.12 |
Cause of death | ||||
Head trauma | 36 (43.9%) | 19 (43.2%) | 9 (40.9%) | 1.00 |
Anoxia | 26 (31.7%) | 19 (43.2%) | 8 (36.4%) | 0.45 |
Other | 20 (24.1%) | 5 (13.3%) | 6 (21.7%) | 0.36 |
Continuous data are shown as means ± standard deviation (SD). PGD, primary graft dysfunction; BMI, body mass index; BSA, body surface area; CKD, chronic kidney disease; VV-ECMO, venovenous extra corporeal membrane oxygenation; ILD; interstitial lung disease; COPD, chronic obstructive pulmonary disease; ARDS, acute respiratory distress syndrome; COVID-19, coronavirus disease 2019; PAH, pulmonary arterial hypertension; CF, cystic fibrosis; WBC, white blood cell; BUN, blood urea nitrogen; AST, aspartate aminotransferase; ALT, Alanine aminotransferase; INR, international normalized ratio
Intra-operative outcomes of lung transplant recipients
Patients with the PGD grade 1 to 3 had longer operative time than the grade 0 patients (6.8±1.5 hours in grade 0 vs. 8.0±1.6 hours in grade 1 or 2 vs. 8.3±1.7 hours in grade 3, P<0.001). The grade 1 or 2 patients had longer ischemic time than grade 0 patients (4.7±1.2 hours in grade 0 vs. 5.3±1.2 hours in grade 1 or 2, P<0.01). The PGD grade 3 group had high intraoperative blood transfusion volumes for all packed red blood cells, fresh frozen plasm, and platelets (P<0.001). Intraoperative veno-arterial ECMO (VA-ECMO) was used more frequently in PGD grades 1 to 3 (grade 0 vs. 1 or 2 vs. 3, 45.8% vs. 82.2% vs. 82.6%, P<0.001). However, the length of VA-ECMO time did not impact the developing any PGD (P=0.74) (Table 2).
Table 2
Variable | PGD grade 0 (n=83) | PGD grade 1/2 (n=45) | PGD grade 3 (n=23) | P value |
---|---|---|---|---|
Intra-operative outcomes | ||||
Operative time (hours) | 6.8±1.5 | 8.0±1.6 | 8.3±1.7 | <0.001 |
Intra-op blood transfusion; pRBC | 1.6±2.9 | 2.8±4.3 | 6.6±5.6 | <0.001 |
Intra-op blood transfusion; FFP | 0.4±1.3 | 1.0±2.0 | 2.4±3.3 | <0.001 |
Intra-op blood transfusion; Plt | 0.4±1.1 | 0.4±0.9 | 1.7±2.2 | <0.001 |
Ischemic time (hours) | 4.7±1.2 | 5.3±1.2 | 5.0±0.9 | <0.01 |
VA-ECMO use | 38 (45.8%) | 37 (82.2%) | 19 (82.6%) | <0.001 |
VA-ECMO time (hours) | 3.0±1.0 | 3.2±0.9 | 3.1±1.2 | 0.74 |
Post-operative outcomes | ||||
AKI | 23 (27.7%) | 19 (42.2%) | 20 (87.0%) | <0.001 |
Dialysis | 3 (3.6%) | 3 (6.7) | 17 (73.9%) | <0.001 |
Stroke | 3 (3.6%) | 1 (2.2%) | 2 (8.7%) | 0.42 |
Ischemic stroke | 2 (2.4%) | 1 (2.2%) | 2 (8.7%) | 0.29 |
Hemorrhagic stroke | 1 (1.2%) | 0 (0.0%) | 0 (0.0%) | 0.66 |
Bowel ischemia | 0 (0.0%) | 0 (0.0%) | 2 (8.7%) | <0.01 |
Digital ischemia | 1 (1.2%) | 1 (2.2%) | 4 (17.4%) | <0.01 |
ICU stay (days) | 8.6±6.7 | 21.0±17.0 | 22.3±14.7 | <0.001 |
Post transplant ventilator (days) | 2.6±5.0 | 8.1±11.4 | 13.4±14.3 | <0.001 |
Hospital stay (days) | 19.8±19.9 | 27.2±18.6 | 45.2±40.8 | <0.001 |
1-year survival rate | 94.0% | 93.1% | 65.2% | <0.01 |
Continuous data are shown as means ± standard deviation (SD) or n (%). PGD, primary graft dysfunction; pRBC, packed red blood cell; FFP, fresh frozen plasma; Plt, platelets; VA-ECMO, venoarterial extra corporeal membrane oxygenation; AKI, acute kidney injury; ICU, intensive care unit.
Post-operative complications and survival of lung transplant recipients
Comparing complications after LT, the incidence of AKI and the use of dialysis were also much higher in grade 3 patients compared to PGD grade 0 and grade 1 or 2 (grade 0 vs. 1 or 2 vs. 3, AKI, 27.7% vs. 42.2% vs. 87.0%, P<0.01; dialysis use, 3.6% vs. 6.7% vs. 73.9%, P<0.001; respectively). The PGD grade 3 group had a higher incidence of digital and intestinal ischemic events to the other groups and the longer duration of ventilator use and ICU stay. The hospital stays in the PGD grade 3 group were longer than in the grade 0 group (Table 2).
In Kaplan-Meier analysis, the survival rate of PGD grade 3 was significantly lower than that of the other two groups (P=0.003) (Figure 1A). Similarly, when compared with the combined group of the grade 0 to 2 patients, the survival rate of the PGD grade 3 group was found to be significantly lower than the other groups (P=0.0006) (Figure 1B).
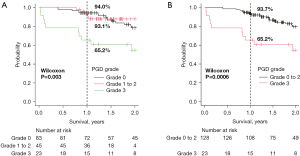
Univariate and multivariate logistic regression analysis of association grade 1 or 2 PGD, and mortality in patients who underwent lung transplants
All variables were placed in a univariate analysis in the cohort without PGD grade 3 patients to identify the risk factors for PGD grade 1 or 2 (Table S1). Bilateral lung transplant cases, etiology of COVID-19 associated ARDS, and pulmonary arterial hypertension (PAH) were more common in PGD grade 1 and 2 groups. Operative time and ischemic time were significantly longer in PGD grade 1 or 2 group than PGD grade 0 group (operative time, OR =1.65, 95% CI: 1.27–2.15, P<0.001; ischemic time, OR =1.59, 95% CI: 1.15–2.19, P<0.01, respectively). Intraoperative VA-ECMO was used in PGD grade 1 or 2 more frequently. Multivariate analysis found that only intraoperative VA-ECMO use was an independent predictive factor of PGD grade 1 or 2 (OR =3.29, 95% CI: 1.00–10.8, P=0.049) (Table 3).
Table 3
Variable | OR | P value | 95% CI |
---|---|---|---|
Recipient factors | |||
Age, years | 0.98 | 0.47 | 0.94–1.03 |
Bilateral | 1.33 | 0.72 | 0.28–6.34 |
Etiology | |||
PAH | 2.54 | 0.12 | 0.78–8.23 |
Laboratory | |||
WBC, 1,000/mm3 | 0.89 | 0.14 | 0.77–1.04 |
Total bilirubin, mg/dL | 0.57 | 0.35 | 0.17–1.88 |
Arterial blood gas | |||
PaO2 | 1.00 | 0.77 | 0.996–1 |
Donor factors | |||
Female | 0.80 | 0.66 | 0.29–2.22 |
Surgical factors | |||
Operative time (hours) | 1.24 | 0.44 | 0.72–2.15 |
Intra-op blood transfusion; pRBC | 0.93 | 0.57 | 0.74–1.18 |
Intra-op blood transfusion; FFP | 1.17 | 0.45 | 0.78–1.75 |
Ischemic time (hours) | 0.92 | 0.76 | 0.53–1.59 |
VA-ECMO use | 3.29 | 0.049 | 1.00–10.8 |
PGD, primary graft dysfunction; OR, odds ratio; CI, confidence interval; PAH, pulmonary arterial hypertension; WBC, white blood cell; pRBC, packed red blood cell; FFP, fresh frozen plasma; VA-ECMO, venoarterial extra corporeal membrane oxygenation.
Univariate and multivariate logistic regression analysis of association grade 3 PGD, and mortality in patients who underwent lung transplants
All variables were placed in a univariate analysis in the cohort without PGD grade 1 or 2 patients to identify the risk factors for PGD grade 3 (Table S2). The results showed that the PGD grade 3 group had significantly younger recipients, more frequent preoperative use of VV-ECMO, more COVID-19 associated ARDS, lower hemoglobin, platelets, and albumin; and higher BUN and total bilirubin in laboratory tests, lower PaO2 in arterial blood gas analysis, longer operative time and ischemic time, higher blood transfusion use, and more frequent intraoperative use of VA-ECMO. Multivariate analysis didn’t find out independent predictive factors of PGD grade 3 (Table 4). In univariate and multivariate analyses between PGD grade 0 and PGD grade 1–3 groups performed as sensitivity analyses, operative time was an independent predictor of PGD occurrence (Tables S3,S4). These results were similar to those of the previous two main cohort analyses.
Table 4
Variable | OR | P value | 95% CI |
---|---|---|---|
Recipient factors | |||
Age, years | 0.97 | 0.43 | 0.91–1.04 |
Pre VV-ECMO use | 95.2 | 0.07 | 0.69–13100 |
Bilateral | 0.12 | 0.12 | 0.01–1.73 |
Etiology | |||
ARDS (COVID-19) | 0.04 | 0.12 | 0.001–2.17 |
Laboratory | |||
Hemoglobin, g/dL | 1.28 | 0.20 | 0.88–1.85 |
Platelets, 1,000/mm3 | 1.00 | 0.91 | 0.99–1.01 |
Sodium, mEq/L | 1.02 | 0.86 | 0.78–1.34 |
BUN, mg/dL | 1.04 | 0.37 | 0.95–1.15 |
Albumin, g/dL | 0.24 | 0.09 | 0.04–1.27 |
Total bilirubin, mg/dL | 0.91 | 0.85 | 0.35–2.39 |
Arterial blood gas | |||
PaO2 | 1.00 | 0.59 | 0.995–1.01 |
Surgical factors | |||
Operative time (hours) | 2.34 | 0.07 | 0.92–5.96 |
Intra-op blood transfusion; pRBC | 0.94 | 0.77 | 0.61–1.44 |
Intra-op blood transfusion; FFP | 0.97 | 0.92 | 0.57–1.65 |
Intra-op blood transfusion; Plt | 1.28 | 0.34 | 0.78–2.09 |
VA-ECMO use | 2.93 | 0.20 | 0.56–15.5 |
PGD, primary graft dysfunction; OR, odds ratio; CI, confidence interval; VV-ECMO, venovenous extra corporeal membrane oxygenation; ARDS, acute respiratory distress syndrome; COVID-19, coronavirus disease 2019; BUN, blood urea nitrogen; pRBC, packed red blood cell; FFP, fresh frozen plasma; Plt, platelets; VA-ECMO, venoarterial extra corporeal membrane oxygenation.
Association of surgery with postoperative complications divided by operative time
The ROC curve analysis showed that 8.18 hours was the cut-off value (AUC =0.70) for the risk of PGD grade 3 (Figure S1). Based on the results of the ROC analysis, the cohort was divided into two groups: those with an operative time of less than 8.18 hours; and those with an operative time of more than 8.18 hours. The higher incidence of PGD grade 3, AKI, dialysis use, and digital ischemia were also more common in the longer operative group (Table S5).
Discussion
Several previous studies reported that various factors were involved in the development of PGD (10-26). On the donor side, factors such as age, smoking history, and alcohol consumption are associated with the risk of developing PGD (10). Recipient factors such as gender, race, obesity, sarcoidosis as the primary disease, and primary hypertension were risk factors (10-20). Surgical factors include single LT, use of intraoperative cardiopulmonary bypass (CPB), blood transfusion, and FiO2 during reperfusion (10). Donor genetic factors such as NADPH oxidase (21) and recipient genetic factors such as polymorphisms in pentraxin-3 (22,23), NFE2L2 (21), prostaglandin 2 (24), Toll interacting protein (25), and IL-17 receptor (26) have also been studied. Most of these reports focused on PGD grade 3, with PGD grade 1 or 2 being considered as a control group or excluded from the cohort. However, it is not clear whether PGD grade 1 or 2 is essentially the same disease group as PGD grade 3, and has same outcomes compared to PGD grade 3. In this study, we sought to demonstrate the differences and similarities between PGD grade 1 or 2 and PGD grade 3 by examining risk factors for each severity of PGD, and to identify factors that determine postoperative outcomes.
The results of univariate analysis showed that bilateral lung transplant cases, etiology of COVID-19 associated ARDS, and PAH, operative time, ischemic time and intraoperative VA-ECMO use may be risk factors affecting PGD grade 1 or 2. On the other hand, recipient age, preoperative VV-ECMO use, bilateral lung transplant cases, etiology of COVID-19 associated ARDS, hemoglobin count, platelet count, BUN, albumin, total bilirubin, PaO2 levels on the day of transplant, operative time, intraoperative blood transfusion volume, and intraoperative VA-ECMO use could be related to PGD grade 3. Multivariate analysis showed that intraoperative VA-ECMO use was an independent risk factor for the development of PGD grade 1 or 2, but no significant independent risk factor for the development of PGD grade 3 was found. It was possible that the multivariate analysis of PGD grade 3 did not show significant results due to the small number of patients in the cohort compared to the number of variables. Our study showed that PGD grade 1 or 2 had the similar risk factors as PGD grade 3, including operative time. The fact that factors that differed significantly from each other were not calculated possibly indicates that PGD grade 1 or 2 and PGD grade 3 are a constant disease group with common risk factors.
In this study, intraoperative factors such as longer operative time and higher blood transfusion volume, intraoperative VA-ECMO use were associated with the development of PGD. Operative time was likely correlated with ischemic time, supporting previous reports that prolonged ischemic time is associated with PGD (20).
Blood transfusion volume may be associated with blood loss, operative time, and use of VA-ECMO during LT. In this study, higher red blood cell transfusions showed an association with a higher grade of PGD. Actually, the ISHLT recommends minimizing blood transfusion during LT. Mechanical circulatory supports are sometimes required due to hypoxemia, right ventricular dysfunction, pulmonary hypertension, and anatomical difficulties. The traditional support has been using full CPB; but in recent years, ECMO has been used instead of CPB. ECMO has been shown to result in less intraoperative transfusion during LT compared with CPB, which may contribute to a reduction in blood transfusion use (27). Based on these findings, we applied central VA-ECMO instead of CPB to all patients in this study cohort.
Once PGD develops, the risk of developing AKI increases dramatically. And the length of ICU stays, postoperative ventilator use, and hospital stay are prolonged. These findings are consistent with previous reports (20). One-year survival was also significantly lower in the PGD grade 3 group, and the Kaplan Meier curve confirmed that early postoperative mortality was high. This result demonstrated the significant impact that PGD grade 3 has on clinical outcomes in the first year after LT.
We calculated that the high-risk group of PGD grade 3 could be extracted most effectively when the operative time was divided by 8.18 hours. In the group with longer operation time, PGD grade 3 occurred in 31.4% of patients; and the risk of postoperative complications of postoperative AKI and digital ischemia was also significantly higher. However, operative time depends not only on the surgical technique but also on various factors such as the use of mechanical circulatory support and the severity of adhesions (28). In fact, patients who were supported by VA-ECMO during lung transplant procedures had significantly longer operative times in our cohort (the data is not shown in the result; non-VA-ECMO 6.2±1.4 hours, VA-ECMO 8.1±1.4 hours, P<0.001). Therefore, this study is not a recommendation to simply reduce operative time. Further studies are needed to clarify the relationship between these factors that can increase operative time and PGD.
There are several limitations to this study. Firstly, this study was single centered, relatively small, and retrospective study in nature. As a result, statistical errors need to be considered. In addition, due to the small number of cases, it was not possible to examine grade 1 and 2 separately. Secondly, there is the potential that unmeasured confounding or bias secondary to missing data limited our results. Our ischemia time was the sum of cold and warm ischemia. If we had been able to separate them, we could have examined them in more detail. Furthermore, there was a lack of information regarding a history of pleurodesis. This factor can also potentially increase operative time and blood loss. Thirdly, we could not examine the long-term postoperative results due to insufficient follow-up periods. In addition, the number of all-cause mortality events was low at 38 (25.2%); and we were unable to examine disease-specific mortality.
Conclusions
In summary, we identified risk factors for the development of PGD grade 1 to 3 after LT and found that the attributable mortality of PGD grade 3 is high in LT. It was indicated that long operative time was associated with PGD and may have influenced postoperative complications.
Acknowledgments
The authors would like to thank Ms. Elena Susan and Mrs. Kweli Pryor for administrative assistance in the submission of this manuscript. This manuscript was presented at the 42nd Annual International Society for Heart and Lung Transplantation Meeting, April 27-30, 2022, Boston, USA.
Funding: AB is supported by National Institutes of Health (No. HL145478, HL147290, and HL147575).
Footnote
Reporting Checklist: The authors have completed the STROBE reporting checklist. Available at https://jtd.amegroups.com/article/view/10.21037/jtd-22-974/rc
Data Sharing Statement: Available at https://jtd.amegroups.com/article/view/10.21037/jtd-22-974/dss
Peer Review File: Available at https://jtd.amegroups.com/article/view/10.21037/jtd-22-974/prf
Conflicts of Interest: All authors have completed the ICMJE uniform disclosure form (available at https://jtd.amegroups.com/article/view/10.21037/jtd-22-974/coif). AB is supported by National Institutes of Health (No. HL145478, HL147290, and HL147575). The other authors have no conflicts of interest to declare.
Ethical Statement: The authors are accountable for all aspects of the work in ensuring that questions related to the accuracy or integrity of any part of the work are appropriately investigated and resolved. This study was approved by the Northwestern University Institutional Review Board (No. STU00207250). The need for patient consent for data collection was waived by the Institutional Review Board as this was a retrospective study. The study was conducted in accordance with the Declaration of Helsinki (as revised in 2013).
Open Access Statement: This is an Open Access article distributed in accordance with the Creative Commons Attribution-NonCommercial-NoDerivs 4.0 International License (CC BY-NC-ND 4.0), which permits the non-commercial replication and distribution of the article with the strict proviso that no changes or edits are made and the original work is properly cited (including links to both the formal publication through the relevant DOI and the license). See: https://creativecommons.org/licenses/by-nc-nd/4.0/.
References
- Christie JD, Carby M, Bag R, et al. Report of the ISHLT Working Group on Primary Lung Graft Dysfunction part II: definition. A consensus statement of the International Society for Heart and Lung Transplantation. J Heart Lung Transplant 2005;24:1454-9. [Crossref] [PubMed]
- Snell GI, Yusen RD, Weill D, et al. Report of the ISHLT Working Group on Primary Lung Graft Dysfunction, part I: Definition and grading-A 2016 Consensus Group statement of the International Society for Heart and Lung Transplantation. J Heart Lung Transplant 2017;36:1097-103. [Crossref] [PubMed]
- Daud SA, Yusen RD, Meyers BF, et al. Impact of immediate primary lung allograft dysfunction on bronchiolitis obliterans syndrome. Am J Respir Crit Care Med 2007;175:507-13. [Crossref] [PubMed]
- Huang HJ, Yusen RD, Meyers BF, et al. Late primary graft dysfunction after lung transplantation and bronchiolitis obliterans syndrome. Am J Transplant 2008;8:2454-62. [Crossref] [PubMed]
- Bellomo R, Ronco C, Kellum JA, et al. Acute renal failure - definition, outcome measures, animal models, fluid therapy and information technology needs: the Second International Consensus Conference of the Acute Dialysis Quality Initiative (ADQI) Group. Crit Care 2004;8:R204-12. [Crossref] [PubMed]
- Kurihara C, Walter JM, Karim A, et al. Feasibility of Venovenous Extracorporeal Membrane Oxygenation Without Systemic Anticoagulation. Ann Thorac Surg 2020;110:1209-15. [Crossref] [PubMed]
- Tomasko J, Prasad SM, Dell DO, et al. Therapeutic anticoagulation-free extracorporeal membrane oxygenation as a bridge to lung transplantation. J Heart Lung Transplant 2016;35:947-8. [Crossref] [PubMed]
- Kurihara C, Walter JM, Singer BD, et al. Extracorporeal Membrane Oxygenation Can Successfully Support Patients With Severe Acute Respiratory Distress Syndrome in Lieu of Mechanical Ventilation. Crit Care Med 2018;46:e1070-3. [Crossref] [PubMed]
- Kanda Y. Investigation of the freely available easy-to-use software 'EZR' for medical statistics. Bone Marrow Transplant 2013;48:452-8. [Crossref] [PubMed]
- Diamond JM, Lee JC, Kawut SM, et al. Clinical risk factors for primary graft dysfunction after lung transplantation. Am J Respir Crit Care Med 2013;187:527-34. [Crossref] [PubMed]
- Liu Y, Liu Y, Su L, et al. Recipient-related clinical risk factors for primary graft dysfunction after lung transplantation: a systematic review and meta-analysis. PLoS One 2014;9:e92773. [Crossref] [PubMed]
- Christie JD, Kotloff RM, Pochettino A, et al. Clinical risk factors for primary graft failure following lung transplantation. Chest 2003;124:1232-41. [Crossref] [PubMed]
- Whitson BA, Nath DS, Johnson AC, et al. Risk factors for primary graft dysfunction after lung transplantation. J Thorac Cardiovasc Surg 2006;131:73-80. [Crossref] [PubMed]
- Burton CM, Iversen M, Milman N, et al. Outcome of lung transplanted patients with primary graft dysfunction. Eur J Cardiothorac Surg 2007;31:75-82. [Crossref] [PubMed]
- Krenn K, Klepetko W, Taghavi S, et al. Recipient vascular endothelial growth factor serum levels predict primary lung graft dysfunction. Am J Transplant 2007;7:700-6. [Crossref] [PubMed]
- Kuntz CL, Hadjiliadis D, Ahya VN, et al. Risk factors for early primary graft dysfunction after lung transplantation: a registry study. Clin Transplant 2009;23:819-30. [Crossref] [PubMed]
- Kreisel D, Krupnick AS, Puri V, et al. Short- and long-term outcomes of 1000 adult lung transplant recipients at a single center. J Thorac Cardiovasc Surg 2011;141:215-22. [Crossref] [PubMed]
- Fang A, Studer S, Kawut SM, et al. Elevated pulmonary artery pressure is a risk factor for primary graft dysfunction following lung transplantation for idiopathic pulmonary fibrosis. Chest 2011;139:782-7. [Crossref] [PubMed]
- Samano MN, Fernandes LM, Baranauskas JC, et al. Risk factors and survival impact of primary graft dysfunction after lung transplantation in a single institution. Transplant Proc 2012;44:2462-8. [Crossref] [PubMed]
- Moon S, Park MS, Lee JG, et al. Risk factors and outcome of primary graft dysfunction after lung transplantation in Korea. J Thorac Dis 2016;8:3275-82. [Crossref] [PubMed]
- Cantu E, Shah RJ, Lin W, et al. Oxidant stress regulatory genetic variation in recipients and donors contributes to risk of primary graft dysfunction after lung transplantation. J Thorac Cardiovasc Surg 2015;149:596-602. [Crossref] [PubMed]
- Diamond JM, Meyer NJ, Feng R, et al. Variation in PTX3 is associated with primary graft dysfunction after lung transplantation. Am J Respir Crit Care Med 2012;186:546-52. [Crossref] [PubMed]
- Diamond JM, Lederer DJ, Kawut SM, et al. Elevated plasma long pentraxin-3 levels and primary graft dysfunction after lung transplantation for idiopathic pulmonary fibrosis. Am J Transplant 2011;11:2517-22. [Crossref] [PubMed]
- Diamond JM, Akimova T, Kazi A, et al. Genetic variation in the prostaglandin E2 pathway is associated with primary graft dysfunction. Am J Respir Crit Care Med 2014;189:567-75. [Crossref] [PubMed]
- Cantu E, Suzuki Y, Diamond JM, et al. Protein Quantitative Trait Loci Analysis Identifies Genetic Variation in the Innate Immune Regulator TOLLIP in Post-Lung Transplant Primary Graft Dysfunction Risk. Am J Transplant 2016;16:833-40. [Crossref] [PubMed]
- Somers J, Ruttens D, Verleden SE, et al. Interleukin-17 receptor polymorphism predisposes to primary graft dysfunction after lung transplantation. J Heart Lung Transplant 2015;34:941-9. [Crossref] [PubMed]
- Magouliotis DE, Tasiopoulou VS, Svokos AA, et al. Extracorporeal membrane oxygenation versus cardiopulmonary bypass during lung transplantation: a meta-analysis. Gen Thorac Cardiovasc Surg 2018;66:38-47. [Crossref] [PubMed]
- Kurihara C, Manerikar A, Querrey M, et al. Clinical Characteristics and Outcomes of Patients With COVID-19-Associated Acute Respiratory Distress Syndrome Who Underwent Lung Transplant. JAMA 2022;327:652-61. [Crossref] [PubMed]