MicroRNA-186-5p inhibits H9c2 cells apoptosis induced by oxygen-glucose deprivation by targeting ERK1/2
Highlight box
Key findings
• MicroRNA-186-5p may suppress oxygen-glucose deprivation-induced apoptosis in H9c2 cells by targeting the ERK1/2 pathway.
What is known and what is new?
• It has been reported that serum MicroRNA-186-5p levels were increased in acute myocardial infarction patients and might contribute to assessing their prognosis.
• ERK1/2 may serve as the negative regulatory target of microRNA-186-5p. MicroRNA-186-5p may inhibit the expression and activation of ERK1/2 and suppress apoptosis in oxygen-glucose deprivation–treated H9c2 cells.
What is the implication, and what should change now?
• The present study suggests that miR-186-5p may be used as a protective factor during acute myocardial ischemia and provides a new theoretical basis for the treatment of this disease.
Introduction
Acute myocardial infarction (AMI) is caused by massive myocardial ischemia and even necrosis in its branches due to sudden plaque or thrombus blocking coronary vessels (1). The process of myocardial infarction involves multiple processes, such as apoptosis, necrosis, oxidative stress, and inflammatory response of myocardial and interstitial cells (2,3). Extensive apoptosis of cardiomyocytes causes ventricular remodeling and even heart failure, and the inhibition of cardiomyocyte apoptosis might effectively preserve cardiac function (2,3).
MicroRNAs (miRNAs) could bind to the 3'-untranslated region (3'-UTR) of the target mRNA to prevent mRNA translation and influence gene expression at the post-transcriptional level (4,5). Several studies revealed that miRNAs regulated diverse processes of cardiovascular biology, including the development of the heart, maintenance of cardiac function, and regulation of cardiomyocyte apoptosis (6-9). Our previous studies demonstrated that serum miR-186-5p levels were increased in AMI patients and might contribute to assessing the prognosis of AMI patients (10,11). However, the underlying molecular mechanism of miR-186-5p in the pathological processes of myocardial ischemia is presently unknown. In this study, we established specific models in vivo and in vitro to investigate the kinetic expression signatures of miR-186-5p. Three bioinformatics databases jointly predicted that ERK1/2 was the potential target protein of miR-186-5p. We performed various biological experiments to verify their targeting relationships and elucidate their biological effects during the process of glucose deficiency and hypoxia. We present the following article in accordance with the ARRIVE reporting checklist (available at https://jtd.amegroups.com/article/view/10.21037/jtd-22-453/rc).
Methods
Animals and AMI operation
A total of 25 specific pathogen-free/Sprague-Dawley (SD) male rats (weighing 250–300 g; 8–10 weeks old) were purchased from the Model Animal Research Center of Jinling Hospital (MARC; China). They were later housed under regular temperature conditions and a 12 h light/dark cycle with the supplement of standard laboratory diet and water ad libitum. Experiments were performed under a project license (No. 2018GKJDWLS-03-056) granted by the Institutional Animal Care and Use Committee of Jinling Hospital in compliance with the institutional guidelines for the Care and Use of Laboratory Animals. The AMI model was induced by ligating the left anterior descending coronary artery (LAD) according to a previously described method (12). SD rats (n=25) were randomly divided into 5 groups using a table of random numbers: the AMI 2 h group (n=5), AMI 4 h group (n=5), AMI 8 h group (n=5), AMI 24 h group (n=5), and the sham-operated group (n=5). The AMI group was treated with descending coronary artery ligation, and the sham-operated group was treated with the same experimental open-chest surgery but without the LAD coronary artery ligation. To confirm that the LAD coronary artery in rats has been successfully ligated, we conducted ECG monitoring during the operation of the AMI model group. When leads II, III, etc., showed the characteristic ST-segment elevation, indicating the presence of myocardial ischemia. To further compare the changes in cardiac structure and histopathology in rats after the experimental operation, the rats were euthanized via anesthesia with 3% pentobarbital at 2, 4, 8, and 24 h after ligation, and the abdominal vein blood and ischemic myocardial tissue samples were taken for further detection. Successful surgery was also determined by observing the status of myocardial tissues after hematoxylin and eosin (H&E) staining (Figure S1).
Cell culture and OGD establishment
H9c2 cells and 293T cells (Chinese Academy of Sciences, Shanghai Cell Bank, China) were cultured in a high-glucose Dulbecco’s Modified Eagle’s Medium (DMEM) (Gibco, USA) containing 10% Fetal Bovine Serum (FBS; Gibco) and 1% penicillin-streptomycin mixture (Gibco) at 37 ℃ in a humidified 5% CO2 atmosphere. Oxygen-glucose deprivation (OGD) model was used to simulate the myocardial ischemic injury in vitro. H9c2 cells were cultured in serum-free and glucose-free DMEM medium in the AnaeroPack-Anaero bag (Mitsubishi Gas Chemical, Japan) and sealed incubator (Mitsubishi Gas Chemical) (13,14). The AnaeroPack-Anaero bag could support an environment of less than 0.1% of oxygen and more than 15% of CO2. Four experimental groups were set up: the OGD 4 h group, OGD 8 h group, OGD 12 h group, and control group.
Cell transfection
H9c2 cells were seeded in 6-well plates and grown to 50–60% confluence before transfection. Lipofectamine 3000 (Thermo, USA) was used to transfect the miR-186-5p mimic/mimic negative control (NC; RiboBio, China) and miR-186-5p inhibitor/inhibitor NC (RiboBio) for 24 h, following the manufacturer’s instruction. The transfection concentration of mimic/mimic NC of miR-186-5p was 100 Nm, and the transfection concentration of inhibitor/inhibitor NC of miR-186-5p was 200 Nm. Then, cells were treated with OGD or not for 8 h. The effect of the synthesized mimic/inhibitor was verified by detecting the levels of miR-186-5p. The regulatory effect of miR-186-5p on the target gene was evaluated by detecting the levels of the protein produced by the target gene.
RNA extraction, reverse transcription, and quantitative real-time polymerase chain reaction (qRT-PCR) analysis
Using TRIzol (Invitrogen, CA, USA) to extract RNA from H9c2 cells. As previously described, the phenol-chloroform purification method was applied to extract RNA from the H9c2 cells and culture medium (15). Total RNA (2 µL) was used to synthesize complementary DNA through reverse transcription in a reaction volume of 10 µL by an avian myeloblastosis virus (AMV) reverse transcriptase system (Takara, Japan) using TaqMan primer (Applied Biosystems, USA) set for miRNAs, as previously described (16). qRT-PCR was performed according to the protocol provided by the manufacturer of TaqMan miRNA probes (Applied Biosystems). U6 served as an internal reference for H9c2 cell samples, and MIR2911 was used for RNA extraction efficiency normalization in the cell culture medium. Relative levels of miR-186-5p were analyzed using the 2−ΔCq method. ΔCq was calculated by subtracting the Cq values of U6 or MIR2911 from that of miR-186-5p.
Western blot analyses
Western blot analysis was performed to detect the expression of related proteins in rats’ myocardial tissues and H9c2 cells. Total protein was extracted according to the instructions provided with protein extraction reagent (Invent, China) and RIPA lysis buffer (Beyotime, China), and quantitative analysis was conducted using a Bradford kit (Beyotime). The protein sample was electrophoresed through SDS-PAGE (5% stacking gel and 10% resolving gel) and transferred onto polyvinylidene fluoride (PVDF) membranes (Bio-Rad, America), and subsequently blocked by 5% nonfat milk dissolved in Tris Buffered Saline with Tween 20 (TBST) for 2 h. After primary antibodies against ERK1/2 (1:1,000; CST, USA), p-ERK1/2 (1:1,000; CST), Cleaved caspase-3 (1:1,000; CST), GAPDH (1:1,000; CST) at 4 ℃ overnight, membranes were washed and incubated with the appropriate secondary horseradish peroxidase-labeled (HRP) antibody at room temperature for 1 h (1:5,000; Santa Cruz, America). GAPDH was used as an internal control. The bands were detected by an ECL kit (Beyotime, China), and band intensity was quantified by Image-J software.
Luciferase reporter assay
Luciferase reporter assay was performed to identify whether ERK1/2 was a target gene of miR-186-5p. The Pmir-REPORT (GenScript, China) plasmid (Figure S2) containing wild-type or mutated binding sites of ERK1/2 3'-UTR fragments (Table S1). The β-galactosidase (β-gal) reporter gene plasmid (Figure S3) was used as the internal control. The 293T cells were seeded into 24-well plates for 24 h. Then, we used lipo-2000 to co-transfected the miR-186-5p mimic/mimic NC/inhibitor/inhibitor NC, ERK1/2 wild-type (WT)/mutator (MUT) plasmid and β-gal plasmid. After 48 h, the luciferase activities were analyzed using the dual-luciferase kit (Promega, America) according to the manufacturer’s protocol. Relative Luciferase activity was the firefly/β-gal luciferase activity ratio.
Apoptosis test
H9c2 cells were seeded into 6-well plates treated with OGD for 8 h. Subsequently, we changed the complete medium and transfected it with miR-186-6p mimic/mimic NC/inhibitor/inhibitor NC for 12 h. Eight groups were set up: the miR-186-5p mimic group; miR-186-5p mimic NC group; MiR-186-5p inhibitor group; miR-186-5p inhibitor NC group; OGD 8 h and miR-186-5p mimic group; OGD 8 h and miR-186-5p mimic NC group; OGD 8 h and miR-186-5p inhibitor group; and OGD 8 h and miR-186-5p inhibitor NC group. Annexin V-FITC/PI kit (Beyotime, China) combined with flow cytometry was used to assess cell apoptosis according to the manufacturer’s instructions. A total of 10,000 cells per group were counted to assess the number of positively-stained cells.
Cell counting kit-8 (CCK-8) assay
The CCK-8 kit (DOJINDO, Japan) was used to detect the regulatory effect of miR-186-5p on the proliferation of H9c2 cells after OGD treatment. The experiment was conducted according to the manufacturer’s protocol.
Statistical analyses
Data in this study are expressed as the mean ± standard deviation of values from at least 3 independent experiments conducted in triplicate. Statistical analyses were performed using SPSS 19.0 (IBM Corp, Armonk, NY, USA). The independent samples t test was used to compare results between 2 groups. One-way analysis of variance (ANOVA) with Tukey’s post hoc analysis was used to compare data from more than 2 groups. P values <0.05 were considered statistically significant.
Results
Expression of miR-186-5p in the OGD models
Our previous study demonstrated the different expression patterns of miR-186-5p in the serum and myocardial tissues of AMI rats (11). In the present study, we further constructed an OGD model of H9c2 cells. Through an optical microscope to observe the cellular morphology of H9c2 cells, we confirmed that the OGD model was constructed successfully (Figure S4). qRT-PCR was used to detect the miR-186-5p expression in H9c2 cells and the culture medium after OGD treatment. The miR-186-5p levels were decreased in OGD-treated H9c2 cells at 8 and 12 h. Conversely, the levels of miR-186-5p in the cell medium were found to be significantly increased in a time-dependent manner at 4, 8, and 12 h (Figure 1). Therefore, we preliminarily speculated that miR-186-5p could be passively released from dead cells induced by OGD treatment.
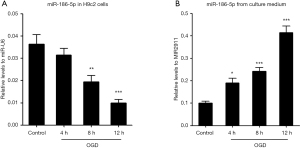
MiR-186-5p targets the 3'UTR of ERK1/2
To further explore the downstream mechanisms of miR-186-5p, 3 bioinformatics databases, including miRanda, PicTar, and TargetScan, were used to predict the possible target genes of miR-186-5p. Additionally, relevant literature was also reviewed. MiR-186-5p might bind to the 3'-UTR of ERK1/2 mRNA (Figure S5).
A luciferase reporter gene assay showed that the luciferase activity of WT-ERK1/2 3'-UTR was significantly decreased after the overexpression of miR-186-5p. In contrast, the luciferase activity of WT-TET2 3'-UTR was found to be significantly increased after the inhibition of miR-186-5p, while no difference was found in MUT-ERK1/2 3'-UTR (Figure 2). The above results indicated that miR-186-5p might specifically bind to the 3'-UTR of ERK1/2 mRNA and inhibit the expression of ERK1/2.
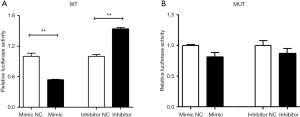
Expression of target and apoptotic protein in the AMI and OGD models
Apoptotic and target protein levels in myocardial tissues of AMI rats were determined by Western blot analyses (Figure 3). Grayscale analyses showed that the level of cleaved caspase-3 was significantly upregulated in myocardial tissues of AMI rats in a time-dependent manner at 4, 8, and 24 h. The target protein t-ERK1/2 and its phosphorylated counterpart p-ERK1/2 were significantly upregulated in myocardial tissues of AMI rats, and the ratio of p-ERK1/2/t-ERK1/2 was also significantly increased, which indicated the activation of the ERK1/2 pathway. We next extracted proteins from different phases of OGD treatment in H9c2 cells for Western blot analyses, respectively. Grayscale analyses showed that OGD treatment promoted the cleaved caspase-3 expression in a time-dependent manner. Additionally, t-ERK1/2 and p-ERK1/2 levels in OGD-treated H9c2 cells were significantly upregulated at 8 and 12 h, respectively, and the ratio of p-ERK1/2/t-ERK1/2 was also significantly increased. The above data indicated the increased apoptosis and activation of the ERK1/2 pathway after AMI and OGD treatment (Figure 4).
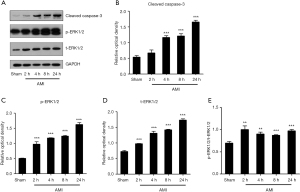
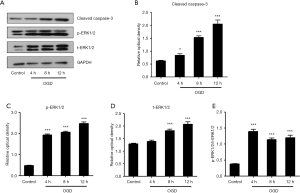
Expression of target proteins and apoptosis protein after the overexpression or inhibition of miR-186-5p
For further study, we transfected the H9c2 cells with the miR-186-5p mimic/NC and miR-186-5p inhibitor/NC. qRT-PCR results indicated that the miR-186-5p mimic significantly increased the expression of miR-186-5p (Figure 5A). Western blot analyses showed that the miR-186-5p mimic could down-regulated the expression of p-ERK1/2 and t-ERK1/2, and the ratio of p-ERK1/2/t-ERK1/2 (Figure 5B-5E). Conversely, the miR-186-5p inhibitor significantly decreased the expression of miR-186-5p (Figure 6A) and could upregulate the expression of p-ERK1/2 and t-ERK1/2, and the ratio of p-ERK1/2/t-ERK1/2 (Figure 6B-6E). Furthermore, the expression of cleaved caspase-3 was found to be decreased in the miR-186-5p mimic group (Figure 7A,7B) but increased in the miR-186-5p inhibitor group (Figure 7C,7D), regardless of whether OGD treatment was performed.
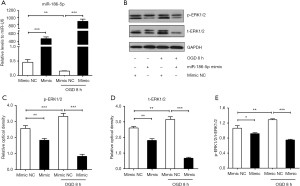
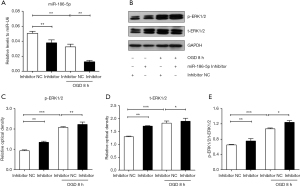
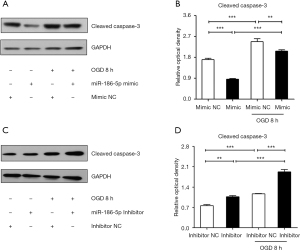
MiR-186-5p inhibited the apoptosis of H9c2 cells in the OGD model
To confirm the effect of miR-186-5p on the apoptosis of H9c2 cells induced by OGD treatment, we performed FCM assays to detect the apoptosis of H9c2 cells after the overexpression and inhibition of miR-186-5p (Figure 8). Compared to mimic/inhibitor NC group, the number of apoptotic cells was significantly decreased in the miR-186-5p mimic group, whereas opposing results were noted in the miR-186-5p inhibitor group. Furthermore, OGD treatment was found to enhance the apoptosis of H9c2 cells.
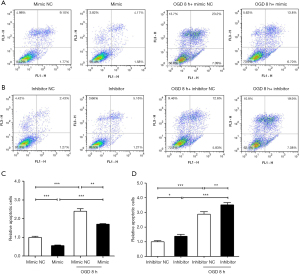
MiR-186-5p promoted the proliferation of H9c2 cells in the OGD model
To further evaluate the effects of miR-186-5p on the proliferation of H9c2 cells induced by OGD treatment, we performed the CCK-8 assays to detect the proliferation of H9c2 cells after the overexpression and inhibition of miR-186-5p (Figure 9). Consequently, the proliferation was significantly increased at 48 and 60 h in the miR-186-5p mimic group and significantly increased at 24, 36, 48, and 60 h in the OGD-treated and miR-186-5p mimic groups, respectively. Meanwhile, the proliferation of the OGD-treated H9c2 cells was found to be significantly increased compared with that of the OGD-untreated H9c2 cells at 60 h after transfecting miR-186-5p mimic.
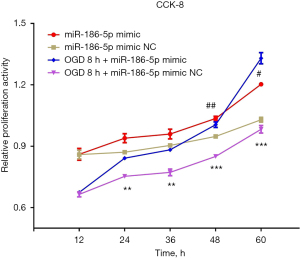
Discussion
In the present study, we found that the level of miR-186-5p was decreased in the OGD-treated H9c2 cells. By using bioinformatics databases analysis and luciferase reporter gene assay, we identified that ERK1/2 might serve as the negative regulatory target of miR-186-5p. Through further transfection experiments, we demonstrated that miR-186-5p might inhibit the expression and activation of ERK1/2, which was also reflected in the reduction of their downstream cleaved caspase-3. Functional experiments helped us to reveal that miR-186-5p may inhibit apoptosis and promote the proliferation of OGD-treated H9c2 cells. Consequently, we concluded that miR-186-5p might suppress OGD-induced apoptosis in H9c2 cells by targeting the ERK1/2 pathway.
MiRNA is a new class of disease biomarkers that can be used to detect biogenesis, including transcription, processing, splicing, export to the cytoplasm, maturation, and binding to the target gene. Our previous study found that the levels of miR-186-5p were increased in the serum of AMI patients and AMI rats (10,11). In the present study, miR-186-5p expression was decreased in H9c2 cells after OGD treatment but increased in the culture medium from the OGD-treated cells. Combining with these aforementioned results, we speculated that miR-186-5p may be passively released from dead cells induced by OGD treatment.
MiRNAs pair with their target mRNA to regulate translation. In mammals, about 60% of all protein-coding genes were controlled by miRNAs (17). In the present study, bioinformatics analysis and luciferase reporter gene assay demonstrated that miR-186-5p could specifically bind to ERK1/2 mRNA 3'-UTR. As a member of the mitogen-activated protein kinase (MAPK) family, ERK1/2 was an important serine-threonine protein kinase, which could be activated by phosphorylation to transduce cell signals to the nucleus in multiple steps. It was mainly involved in cell proliferation, differentiation, and apoptosis (18,19). The MAPK family plays an important role in inflammatory reactions in various diseases, and the ERK1/2-NF-κb pathway affects the activation of the NLRP3 inflammasome in the atherosclerosis process (20). Studies have shown that ERK1/2 could affect myocardial fibrosis after infarction by regulating the proliferation of myocardial fibroblasts (21). ERK1/2 has also been shown to participate in the hyperplasia and hypertrophy of ventricular myocytes after myocardial infarction by influencing the expression of brain natriuretic peptide and c-fos (22). Additionally, inhibition of ERK1/2 activation reduces mitochondrial lysis in H9c2 cells, thereby alleviating myocardial apoptosis induced by reoxygenation after hypoxia (23,24). Therefore, ERK1/2 plays a complex and important role in the hypoxic stress environment of cardiomyocytes.
An increasing number of studies have demonstrated that miRNAs play important roles in cardiogenesis, endothelial injury, and abnormal inflammation by the regulation of apoptosis of cardiomyocytes (25-29). In this study, transfection experiments were further performed to determine the specific role of miR-186-5p in OGD-treated H9c2 cells. Our results suggested that miR-186-5p might inhibit the expression and activation of ERK1/2. This finding was also reflected in the reduction of their downstream apoptosis-related proteins, such as cleaved caspase-3. Flow cytometry assays were performed to reveal that miR-186-5p could attenuate the OGD-induced apoptosis of H9c2 cells. Additionally, the CCK-8 experiments were conducted to clarify that miR-186-5p alleviated the reduced proliferation of H9c2 cells induced by OGD treatment. The studies have shown that miR-186-5p might regulate the proliferation, apoptosis, and inflammatory response of cardiomyocytes by targeting IGF-1 and inhibiting TLR3 (30-34). However, no reports have been found about miR-186-5p targeting ERK1/2 to induce myocardial cell injury caused by glucose deficiency and hypoxia. The present study further identified that miR-186-5p in H9c2 cells might be used as a protective factor to inhibit cell damage caused by ischemia and hypoxia.
Our study had some limitations. First, although this study confirmed the association between miR-186-5p and its target protein and the possible role of miR-186-5p in OGD-treated H9c2 cells, the mechanism of how ERK1/2 affected downstream molecules, such as the pro-caspase3 or the poly (ADP-ribose) polymerase (PARP) to regulate cell apoptosis, was still unknown. Additionally, further experiments are needed to elucidate the underlying signaling pathway and whether the altered apoptotic and proliferative capacity of cardiomyocytes was regulated exclusively by miR-186-5p and ERK1/2. To confirm the effect of miR-186-5p on myocardial ischemia and hypoxia, it is also necessary to construct animal models of overexpression or inhibition of miR-186-5p and evaluate the value of antagonistic myocardial apoptosis or therapeutic in vivo.
Conclusions
The present study demonstrated that miR-186-5p might inhibit the apoptosis and promote the proliferation of H9c2 cells induced by glucose deficiency and hypoxia by targeting ERK1/2. These findings suggest that miR-186-5p can be used as a protective factor during acute myocardial ischemia. This study provides a new theoretical basis for treating this disease.
Acknowledgments
Funding: This work was supported by the National Natural Science Foundation of China (Nos. 81871702; 82172360).
Footnote
Reporting Checklist: The authors have completed the ARRIVE reporting checklist. Available at https://jtd.amegroups.com/article/view/10.21037/jtd-22-453/rc
Data Sharing Statement: Available at https://jtd.amegroups.com/article/view/10.21037/jtd-22-453/dss
Conflicts of Interest: All authors have completed the ICMJE uniform disclosure form (available at https://jtd.amegroups.com/article/view/10.21037/jtd-22-453/coif). The authors have no conflicts of interest to declare.
Ethical Statement: The authors are accountable for all aspects of the work in ensuring that questions related to the accuracy or integrity of any part of the work are appropriately investigated and resolved. Experiments were performed under a project license (No. 2018GKJDWLS-03-056) granted by the Institutional Animal Care and Use Committee of Jinling Hospital in compliance with the institutional guidelines for the Care and Use of Laboratory Animals.
Open Access Statement: This is an Open Access article distributed in accordance with the Creative Commons Attribution-NonCommercial-NoDerivs 4.0 International License (CC BY-NC-ND 4.0), which permits the non-commercial replication and distribution of the article with the strict proviso that no changes or edits are made and the original work is properly cited (including links to both the formal publication through the relevant DOI and the license). See: https://creativecommons.org/licenses/by-nc-nd/4.0/.
References
- Falk E, Nakano M, Bentzon JF, et al. Update on acute coronary syndromes: the pathologists’ view. Eur Heart J 2013;34:719-28. [Crossref] [PubMed]
- Han Y, Cai Y, Lai X, et al. lncRNA RMRP Prevents Mitochondrial Dysfunction and Cardiomyocyte Apoptosis via the miR-1-5p/hsp70 Axis in LPS-Induced Sepsis Mice. Inflammation 2020;43:605-18. [Crossref] [PubMed]
- Wei L, Zhou Q, Tian H, et al. Integrin β3 promotes cardiomyocyte proliferation and attenuates hypoxia-induced apoptosis via regulating the PTEN/Akt/Mtor and ERK1/2 pathways. Int J Biol Sci 2020;16:644-54. [Crossref] [PubMed]
- Mohr AM, Mott JL. Overview of microRNA biology. Semin Liver Dis 2015;35:3-11. [Crossref] [PubMed]
- Bartel DP. MicroRNAs: target recognition and regulatory functions. Cell 2009;136:215-33. [Crossref] [PubMed]
- Wojciechowska A, Braniewska A, Kozar-Kamińska K. MicroRNA in cardiovascular biology and disease. Adv Clin Exp Med 2017;26:865-74. [Crossref] [PubMed]
- Xiao X, Lu Z, Lin V, et al. MicroRNA miR-24-3p Reduces Apoptosis and Regulates Keap1-Nrf2 Pathway in Mouse Cardiomyocytes Responding to Ischemia/Reperfusion Injury. Oxid Med Cell Longev 2018;2018:7042105. [Crossref] [PubMed]
- Ning S, Li Z, Ji Z, et al. MicroRNA 494 suppresses hypoxia/reoxygenation induced cardiomyocyte apoptosis and autophagy via the PI3K/AKT/Mtor signaling pathway by targeting SIRT1. Mol Med Rep 2020;22:5231-42. [Crossref] [PubMed]
- Li AY, Yang Q, Yang K. miR-133a mediates the hypoxia-induced apoptosis by inhibiting TAGLN2 expression in cardiac myocytes. Mol Cell Biochem 2015;400:173-81. [Crossref] [PubMed]
- Wu J, Song J, Wang C, et al. Identification of serum microRNAs for cardiovascular risk stratification in dyslipidemia subjects. Int J Cardiol 2014;172:232-4. [Crossref] [PubMed]
- Li Z, Wu J, Wei W, et al. Association of Serum miR-186-5p With the Prognosis of Acute Coronary Syndrome Patients After Percutaneous Coronary Intervention. Front Physiol 2019;10:686. [Crossref] [PubMed]
- Yang B, Lin H, Xiao J, et al. The muscle-specific microRNA miR-1 regulates cardiac arrhythmogenic potential by targeting GJA1 and KCNJ2. Nat Med 2007;13:486-91. [Crossref] [PubMed]
- Katoh C, Osanai T, Tomita H, et al. Brain natriuretic peptide is released from human astrocytoma cell line U373MG under hypoxia: a possible role in anti-apoptosis. J Endocrinol 2011;208:51-7. [Crossref] [PubMed]
- Kamiya T, Kwon AH, Kanemaki T, et al. A simplified model of hypoxic injury in primary cultured rat hepatocytes. In Vitro Cell Dev Biol Anim 1998;34:131-7. [Crossref] [PubMed]
- Wang C, Wan S, Yang T, et al. Increased serum microRNAs are closely associated with the presence of microvascular complications in type 2 diabetes mellitus. Sci Rep 2016;6:20032. [Crossref] [PubMed]
- Lu P, Wang F, Wu J, et al. Elevated Serum miR-7, miR-9, miR-122, and miR-141 Are Noninvasive Biomarkers of Acute Pancreatitis. Dis Markers 2017;2017:7293459. [Crossref] [PubMed]
- Friedman RC, Farh KK, Burge CB, et al. Most mammalian mRNAs are conserved targets of microRNAs. Genome Res 2009;19:92-105. [Crossref] [PubMed]
- Yang SH, Sharrocks AD, Whitmarsh AJ. Transcriptional regulation by the MAP kinase signaling cascades. Gene 2003;320:3-21. [Crossref] [PubMed]
- Yoon S, Seger R. The extracellular signal-regulated kinase: multiple substrates regulate diverse cellular functions. Growth Factors 2006;24:21-44. [Crossref] [PubMed]
- Yin R, Zhu X, Wang J, et al. MicroRNA-155 promotes the ox-LDL-induced activation of NLRP3 inflammasomes via the ERK1/2 pathway in THP-1 macrophages and aggravates atherosclerosis in ApoE-/- mice. Ann Palliat Med 2019;8:676-89. [Crossref] [PubMed]
- Liu Z, Xu Q, Yang Q, et al. Vascular peroxidase 1 is a novel regulator of cardiac fibrosis after myocardial infarction. Redox Biol 2019;22:101151. [Crossref] [PubMed]
- He Q, Harding P, LaPointe MC. PKA, Rap1, ERK1/2, and p90RSK mediate PGE2 and EP4 signaling in neonatal ventricular myocytes. Am J Physiol Heart Circ Physiol 2010;298:H136-43. [Crossref] [PubMed]
- Zhao L, Zhuang J, Wang Y, et al. Propofol Ameliorates H9c2 Cells Apoptosis Induced by Oxygen Glucose Deprivation and Reperfusion Injury via Inhibiting High Levels of Mitochondrial Fusion and Fission. Front Pharmacol 2019;10:61. [Crossref] [PubMed]
- Li F, Li D, Tang S, et al. Quercetin Protects H9c2 Cardiomyocytes against Oxygen-Glucose Deprivation/Reoxygenation-Induced Oxidative Stress and Mitochondrial Apoptosis by Regulating the ERK1/2/DRP1 Signaling Pathway. Evid Based Complement Alternat Med 2021;2021:7522175. [Crossref] [PubMed]
- Kaur A, Mackin ST, Schlosser K, et al. Systematic review of microRNA biomarkers in acute coronary syndrome and stable coronary artery disease. Cardiovasc Res 2020;116:1113-24. [Crossref] [PubMed]
- Wu S, Sun H, Sun B. MicroRNA-145 is involved in endothelial cell dysfunction and acts as a promising biomarker of acute coronary syndrome. Eur J Med Res 2020;25:2. [Crossref] [PubMed]
- Wang X, Shang Y, Dai S, et al. MicroRNA-16-5p Aggravates Myocardial Infarction Injury by Targeting the Expression of Insulin Receptor Substrates 1 and Mediating Myocardial Apoptosis and Angiogenesis. Curr Neurovasc Res 2020;17:11-7. [Crossref] [PubMed]
- Zhao Z, Du S, Shen S, et al. microRNA-132 inhibits cardiomyocyte apoptosis and myocardial remodeling in myocardial infarction by targeting IL-1β. J Cell Physiol 2020;235:2710-21. [Crossref] [PubMed]
- Yang B, Dong R, Zhao H. Inhibition of microRNA-346 inhibits myocardial inflammation and apoptosis after myocardial infarction via targeting NFIB. Eur Rev Med Pharmacol Sci 2020;24:11752-60. [Crossref] [PubMed]
- Jiang J, Mo H, Liu C, et al. Inhibition of miR-186-5p contributes to high glucose-induced injury in AC16 cardiomyocytes. Exp Ther Med 2018;15:627-32. [Crossref] [PubMed]
- Xu H, Li J, Zhao Y, et al. TNFα-induced downregulation of microRNA-186 contributes to apoptosis in rat primary cardiomyocytes. Immunobiology 2017;222:778-84. [Crossref] [PubMed]
- Liu Y, Zheng W, Pan Y, et al. Low expression of miR-186-5p regulates cell apoptosis by targeting toll-like receptor 3 in high glucose-induced cardiomyocytes. J Cell Biochem 2019;120:9532-8. [Crossref] [PubMed]
- Wang R, Bao H, Zhang S, et al. miR-186-5p Promotes Apoptosis by Targeting IGF-1 in SH-SY5Y OGD/R Model. Int J Biol Sci 2018;14:1791-9. [Crossref] [PubMed]
- Yao Y, Zhang X, Chen HP, et al. MicroRNA-186 promotes macrophage lipid accumulation and secretion of pro-inflammatory cytokines by targeting cystathionine γ-lyase in THP-1 macrophages. Atherosclerosis 2016;250:122-32. [Crossref] [PubMed]
(English Language Editor: C. Mullens)