Immunoproteasomes and immunotherapy—a smoking gun for lung cancer?
Defining a therapeutic window in chemo- and immunotherapy of cancer
An important point in cancer treatment is defining molecular differences between tumors and normal tissue. First line treatment usually involves surgery but when the tumor is not completely removed, radiotherapy and/or chemotherapy are the next possible options. Radiotherapy induces DNA damage, as do many chemotherapy treatments. The concept is that the tumor is more sensitive to DNA damage as it proliferates faster than normal tissue. Other chemotherapeutics affect metabolism and microtubule stability, factors where fast growing tumor cells can be expected to be more sensitive than slower growing healthy cells. Some tumors can have specific mutated drivers that can be targeted by compounds such as the Abl inhibitor (Gleevec) in CML tumors or the BRAF (V600E) inhibitors in melanoma and sometimes (~2% of the cases) NSCLC (1-4).
Both melanoma and NSCLC are tumors specified by many DNA mutations, due to respectively UV light exposure and smoking behavior. This fact can make these tumors more susceptible to the adaptive immune system that is trained to recognize new antigens (such as viruses, transplanted tissue but also mutated antigens; the immune response does not distinguish between these) (5,6). The interesting consequence of this aspect of the adaptive immune surveillance is that tumors with mutated antigens can be less sensitive to chemotherapy but more sensitive to immunotherapy. But how to activate the adaptive immune system against cancer?
Current immunotherapy treatments for various forms of cancer
Tumors are obviously different with respect to their mutation rate (in immunological terms; antigenic load), their expression of MHC class I molecules (that should present the antigenic load to the immune system), their access by the immune system, and their local control of the immune system (the tumor microenvironment). It may not be a surprise that various ways to direct the immune system to a tumor have been studied for decades. As soon as it was realized that MHC class I molecules present peptides of intracellular proteins to the immune system, peptide vaccination, peptide loading on dendritic cells, RNA transfection of antigens, exosome vaccination and more have been tried (7). These are a type of personalized treatment protocol where both the antigen and the immune cells of the patient are being used to try to generate strong immune responses against antigens specific for a tumor. The prime tumor in these studies was melanoma, which is considered immunogenic because of their many point mutations and the expression of proteins otherwise only expressed in melanocytes (such as tyrosinase and gp100), but also because of their spontaneous regression in a small subset of patients. While immune responses were boosted by the various therapies, these yielded responses in only a small set of patients, and the question was whether these responses could be improved (8).
Another approach was to extract lymphocytes from the patient tumor followed by expansion ex vivo (either or not with tumor fragments or peptides) in a medium supplemented with interleukin 2 (IL-2), a cytokine that assist in T cell differentiation and activation. These T cells are subsequently re-infused in the patient in the hope that this induces a stronger anti-tumor response. This is called tumor infiltrating lymphocyte (TIL) therapy (Figure 1). This type of therapy yielded response rates in various trials of approximately 50% in stage IV melanoma patients and 7–20% of the patients in various studies had a complete response and long overall survival (OS) (9,10). TIL therapy has been tested mostly with melanoma patients, as these tumors have been proposed to be most sensitive to immune cell attack. A particular problem with this therapy is that it is labor intensive, immune cell based and extraordinary personalized. For these reasons, the therapy is also very expensive. A therapy where one compound can be used for many patients would be a better and more cost effective option for immunotherapy of tumors.
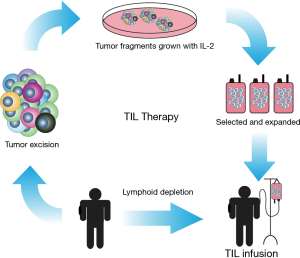
Such an immunotherapy has been developed in cancer for some years. Initially humanized antibodies against unique proteins expressed on tumor cells were introduced in oncology treatment. Examples include Herceptin against Her2/neu in breast cancer and rituximab against CD20 in B cell lymphoma (11,12). These antibodies likely attract the complement system for elimination of the protein expressing tumor cells. These are examples of targeted therapies directed against relatively unique antigens on defined tumors. Alas, there are only few examples of such tumor-specific cell surface expressed proteins.
Accelerating immune responses against the mutations in tumors would be another way to boost immunotherapy of cancer. This was originally done by treating patients with IFNγ or IL-2 with some reported responses in patients (13,14). These studies were not further expanded due to the induced strong auto-immune responses. More recently, antibodies were developed targeting proteins on T cells or (tumor) target cells that normally keep the immune system ‘in check’. These so-called checkpoint antibodies have revolutionized immunotherapy. Humanized antibodies against two checkpoint proteins are currently used in clinical trials; antibodies against programmed cell death protein (PD)-1 (or its ligand PD-L1) and antibodies against CTLA-4. PD-1 is a cell surface protein expressed by T and B cells. It functions as an immune checkpoint by interacting with its ligand PD-L1 or PD-L2 on other cells including various tumor cells (Figure 2). This interaction dampens the activation of the T lymphocytes. The PD-1, PD-L1/2 system is developed to prevent auto-immunity, however, cancer is capable of using this system to its advantage by inactivating the immune response against itself by simply expressing PD-L1 or PD-L2. Immunotherapy using PD-1 blockades is based on interrupting this interaction with specific humanized antibodies and allowing tumor-specific T cells that are kept in check by the tumor environment to now become activated to, hopefully, eliminate the tumor cells, which indeed is successful in some 20% of melanoma patients. This is a prime example of an effective immune checkpoint blockade and successful immunotherapy (15).
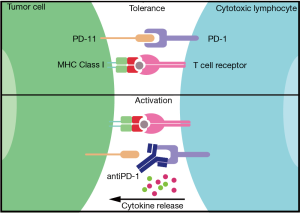
CTLA-4 is a receptor found at the surface of T cells that competes likely with the activating receptor CD28 for the same ligands. CTLA-4 inhibits CD28 thus preventing the activation of lymphocytes, in fact creating immune tolerance. When CTLA-4 control is inhibited (for examples by antibodies, like ipilimumab), cytotoxic T cells can become activated to recognize and destroy cancer cells, when they were recognizing these tumors. Blocking both PD-1 and CTLA4 by combining nivolumab and ipilimumab can further improve immune responses against untreatable melanoma. This showed a significant longer disease-free progression in patients treated with this antibody combination therapy than the single therapy arms and reported a 2-year OS of 40%. Interestingly, a direct link between PD-L1 expression on melanoma tumors and the success of checkpoint antibodies was sometimes observed (16) while another study failed to observe this (17). There is currently not a good, validated and accepted biomarker that predicts successful tumor immunotherapy with checkpoint antibodies. In addition, it should be noted that these therapies come at a price as the immune system is triggered to become auto-reactive. Autoimmune responses following checkpoint antibody therapy are indeed frequently observed (18) and can limit treatment. The question is then to what extent immunotherapy with checkpoint antibodies can be improved to cure more patients while controlling autoimmune responses, but this definitively will be a major area of research for the coming years.
Immunotherapy in non-small cell lung cancer (NSCLC)
If checkpoint antibodies are effective in melanoma because of the many neo-antigens that are seen by cytotoxic T cells as foreign, then these antibodies may be effective in lung cancer as well, as smoking induces many mutations in these tumors (19). In the past year the FDA has approved two humanized anti-PD-1 antibodies for therapeutic use in advanced lung cancer; Keytruda (pembrolizumab) and Opdivo (nivolumab). Three more PD-1 blockade antibodies are currently in phase III trials; atezolizumab, durvalumab and avelumab. These trials are testing various treatment options in combination and as stand-alone treatment.
A pooled analysis study of NSCLC patients combined seven studies on the effect of checkpoint antibodies including 914 patients in total. An objective response of checkpoint antibodies on OS of NSCLC patients was observed. Blocking PD-1/PD-L1 with these antibodies yielded some 24% OS after 2 years (the docetaxel control arm had a 10% survival at that point) (19). Surprisingly, it is unclear whether this relates to PD-1 or PD-L1 expression, as the studies contradict each other on this point (15,20,21). Yet, these studies suggest that the presentation of mutated antigens through MHC class I to cytotoxic T cells that have lost their checkpoint control can be effective for a subgroup of lung cancer patients. The question is, can this be improved to yield responses to a larger group of NSCLC patients. One option could be to improve the presentation of the mutated antigens by MHC class I molecules (16).
The immunoproteasome in support of immunotherapy
Antigen presentation of mutated antigens by MHC class I molecules follow a particular path (22). Proteins are degraded in small fragments by the proteasome. These fragments can be further trimmed or even fully degraded into single amino acids. A fraction of the peptides manage to contact a peptide transporter in the ER membrane, called TAP. TAP introduces the peptides in the ER for consideration by MHC class I that selects high affinity ones for display at the cell surface and consideration by cytotoxic T cells.
The proteasome is a large multisubunit protease in the form of a constricted barrel with three different protease subunits in the middle chamber. Proteins (usually after modification by ubiquitin) have to enter this chamber in an unfolded state for degradation into fragments (i.e., peptides). The proteasome comes in different flavors. The two best known ones are the constitutive and the immunoproteasome (23-25). The three active subunits of the proteasome are replaced by three others in the immunoproteasome (i.e., in immune cells or after cell exposure to IFN-γ) resulting in an altered handling of antigens (Figure 3). In general it is assumed that the immunoproteasome is geared at making peptides that are optimal for antigen presentation by MHC class I molecules, thus resulting in better immune responses. Is this relevant for the immune responses to NSCLC?
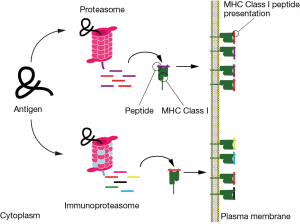
A recent article by Tripathi and colleges (26) investigates the negative regulation of immunoproteasome subunits in NSCLC with a mesenchymal phenotype and how this can be associated to a poor outcome. They reported down regulation of immunoproteasomal subunits in cell lines that have undergone epithelial to mesenchymal (EMT) change together with patient data underlining that the negative regulation of these subunits could serve as a marker for EMT and is associated with poor outcome. They first analyzed gene expression profiles of all 49 genes related to proteasome and associated processes. A significant difference was observed for immunoproteasome subunits and for related genes. These included lower expression of IRF1 and STAT1, proteins involved in regulating expression of immunoproteasome subunits. This related to gene expression profiles corresponding to mesenchymal cell types (27). Further analyses suggested that these expression profiles could serve as a prognostic factor for EMT. Immunohistochemical staining for immunoproteasome subunit PSMB8 corresponded to decreased survival for patients in the lowest quartile of expression. The authors did not report staining for the other two immunoproteasome subunits PSMB9 and PSMB10. A lower mRNA expression of PSMB8 and PSMB9 in recurrent lung tumors was extracted from public datasets. Alas, the authors did not complete the analyses by including PSMB10. Yet expression of immunoproteasomes, definitively in light of the immunotherapy approaches discussed above, could be an interesting prognostic factor, especially when such data could be linked to clinical responses to checkpoint inhibitors. This was not included in this study.
Why would immunoproteasome expression affect clinical outcome? One option is that the peptidome on MHC class I (named HLA in human) in NSCLC is different due to immunoproteasome expression (Figure 3). The authors tested this and identified peptides that are presented on a variety of cell lines in different EMT states. These data suggested that the diversity and numbers of MHC class I presented peptides is much lower in mesenchymal cell lines than in epithelial cell lines. These data were further supported by initiating immunoproteasome expression by simply exposing the mesenchymal cell lines to IFN-γ followed by analysis of the MHC class I peptidome. Differences in immunoproteasome expression in NSCLC may result in different antigen degradation and then a different peptidome presented by MHC class I to cytotoxic T cells. This may promote a better outcome of disease… (26).
And now…
The immunoproteasome does not degrade more antigens but perhaps produces better fragments for presentation by MHC class I. It may, however, be difficult to understand how the immunoproteasome ‘knows’ which fragments are best for the different MHC class I alleles in an outbred population such as Homo sapiens. Yet, it is likely that a more diverse peptide pool from the antigens present in a cell increases the option for presentation of the modified fragment that can be recognized by cytotoxic T cells as non-self. TIL therapy effectiveness has been linked to the diversity of tumor antigens found in melanoma (9). Along these lines, it is possible that the effects of anti-PD-1 or any other checkpoint antibody treatment would also depend on the variability and diversity of the peptide repertoire presented by MHC class I on tumor cells. Immunoproteasome expression levels could then assist in determining the responses to T cells awakened after the removal of their checkpoint by anti-PD-1 antibodies. One recent publication about the genomic and transcriptomic features of patients either or not responding to anti-PD-1 treatment correlated a high mutational load in melanoma with improved survival. This is unlikely the whole story since the same study showed that objective responses did not correlated to these factors (27,28).
These were data for melanoma and the studies on the effect of checkpoint therapy on NSCLC are lagging behind these studies. Yet, Tripathi et al. (26) provide information that can be used in combination with checkpoint inhibitors as they suggest an important role of expression of the immunoproteasome in NSLCL in responsiveness to immunotherapy. How to induce this expression is not further discussed but could be of interest to study with the aim to include this in combination with checkpoint antibody therapy. One option is to combine checkpoint antibodies with radiotherapy where MHC class I expression is increased by mTOR activation resulting in better TIL therapy and checkpoint antibody responses (29,30). Another option may be to combine PD-1 blockades, TIL therapy and IFN-γ: first start with IFN-γ treatment of the patient to induce upregulation of immunoproteasome expression and MHC class I associated peptide diversity. Then remove the tumor and proceed with a TIL therapy protocol. Once the tumor specific cytotoxic T cells have expanded, a further treatment with checkpoint antibodies may allow optimal T cell responses in the tumor microenvironment while overcoming the many local immune suppressive mechanisms. This may further increase the OS of NSCLC patients after immunotherapy. Yet, it may come at a cost; the financial costs of this personalized treatment will be exceedingly high and the therapy may be limited by accelerated autoimmune responses. The advantage of combining checkpoint antibodies with radiotherapy is that the latter is local rather than systemic thus limiting side effects such as auto-immunity responses. Yet these or other ways to induce expression of the immunoproteasome to improve the diversity of MHC class I presented peptide repertoire may be an important step on the road to immunotherapy as a mainstream treatment option for cancer.
Acknowledgements
None.
Footnote
Provenance: This is an invited Perspective commissioned by the Section Editor Long Jiang (Second Affiliated Hospital, Institute of Respiratory Diseases, Zhejiang University School of Medicine, Hangzhou, China).
Conflicts of Interest: The authors have no conflicts of interest to declare.
References
- Howlader N, Noone AM, Krapcho M, et al. SEER Cancer Statistics Review, 1975-2013, National Cancer Institute. Bethesda, MD. Available online: http://seer.cancer.gov/csr/1975_2013/, based on November 2015 SEER data submission, posted to the SEER web site, April 2016.
- Fiorentini G, Rossi S, Lanzanova G, et al. Tyrosine kinase inhibitor imatinib mesylate as anticancer agent for advanced ocular melanoma expressing immunoistochemical C-KIT (CD 117): preliminary results of a compassionate use clinical trial. J Exp Clin Cancer Res 2003;22:17-20. [PubMed]
- Flaherty KT, Puzanov I, Kim KB, et al. Inhibition of mutated, activated BRAF in metastatic melanoma. N Engl J Med 2010;363:809-19. [Crossref] [PubMed]
- Marchetti A, Felicioni L, Malatesta S, et al. Clinical features and outcome of patients with non-small-cell lung cancer harboring BRAF mutations. J Clin Oncol 2011;29:3574-9. [Crossref] [PubMed]
- Campesato LF, Barroso-Sousa R, Jimenez L, et al. Comprehensive cancer-gene panels can be used to estimate mutational load and predict clinical benefit to PD-1 blockade in clinical practice. Oncotarget 2015;6:34221-7. [PubMed]
- Leisegang M, Kammertoens T, Uckert W, et al. Targeting human melanoma neoantigens by T cell receptor gene therapy. J Clin Invest 2016;126:854-8. [Crossref] [PubMed]
- Palucka AK, Coussens LM. The basis of oncoimmunology. Cell 2016;164:1233-47. [Crossref] [PubMed]
- Lee N, Zakka LR, Mihm MC Jr, et al. Tumour-infiltrating lymphocytes in melanoma prognosis and cancer immunotherapy. Pathology 2016;48:177-87. [Crossref] [PubMed]
- Kelderman S, Heemskerk B, Fanchi L, et al. Antigen-specific TIL therapy for melanoma: a flexible platform for personalized cancer immunotherapy. Eur J Immunol 2016. [Epub ahead of print]. [Crossref] [PubMed]
- Hinrichs CS, Rosenberg SA. Exploiting the curative potential of adoptive T-cell therapy for cancer. Immunol Rev 2014;257:56-71. [Crossref] [PubMed]
- Tokuyama H, Hagi T, Mattarollo SR, et al. V gamma 9 V delta 2 T cell cytotoxicity against tumor cells is enhanced by monoclonal antibody drugs--rituximab and trastuzumab. Int J Cancer 2008;122:2526-34. [Crossref] [PubMed]
- Persons DL, Bui MM, Lowery MC, et al. Fluorescence in situ hybridization (FISH) for detection of HER-2/neu amplification in breast cancer: a multicenter portability study. Ann Clin Lab Sci 2000;30:41-8. [PubMed]
- Chianese-Bullock KA, Woodson EM, Tao H, et al. Autoimmune toxicities associated with the administration of antitumor vaccines and low-dose interleukin-2. J Immunother 2005;28:412-9. [Crossref] [PubMed]
- Pollard KM, Cauvi DM, Toomey CB, et al. Interferon-γ and systemic autoimmunity. Discov Med 2013;16:123-31. [PubMed]
- Zheng P, Zhou Z. Human cancer immunotherapy with PD-1/PD-L1 blockade. Biomark Cancer 2015;7:15-8. [PubMed]
- Larkin J, Chiarion-Sileni V, Gonzalez R, et al. Combined nivolumab and ipilimumab or monotherapy in untreated melanoma. N Engl J Med 2015;373:23-34. [Crossref] [PubMed]
- Passiglia F, Bronte G, Bazan V, et al. PD-L1 expression as predictive biomarker in patients with NSCLC: a pooled analysis. Oncotarget 2016. [Epub ahead of print]. [Crossref] [PubMed]
- Zimmer L, Goldinger SM, Hofmann L, et al. Neurological, respiratory, musculoskeletal, cardiac and ocular side-effects of anti-PD-1 therapy. Eur J Cancer 2016;60:210-25. [Crossref] [PubMed]
- Perera D, Poulos RC, Shah A, et al. Differential DNA repair underlies mutation hotspots at active promoters in cancer genomes. Nature 2016;532:259-63. [Crossref] [PubMed]
- Brahmer J, Reckamp KL, Baas P, et al. Nivolumab versus docetaxel in advanced squamous-cell non-small-cell lung cancer. N Engl J Med 2015;373:123-35. [Crossref] [PubMed]
- Gandini S, Massi D, Mandalà M. PD-L1 expression in cancer patients receiving anti PD-1/PD-L1 antibodies: a systematic review and meta-analysis. Crit Rev Oncol Hematol 2016;100:88-98. [Crossref] [PubMed]
- Neefjes J, Jongsma ML, Paul P, et al. Towards a systems understanding of MHC class I and MHC class II antigen presentation. Nat Rev Immunol 2011;11:823-36. [PubMed]
- Ciechanover A, Stanhill A. The complexity of recognition of ubiquitinated substrates by the 26S proteasome. Biochim Biophys Acta 2014;1843:86-96.
- Kuhn DJ, Hunsucker SA, Chen Q, et al. Targeted inhibition of the immunoproteasome is a potent strategy against models of multiple myeloma that overcomes resistance to conventional drugs and nonspecific proteasome inhibitors. Blood 2009;113:4667-76. [Crossref] [PubMed]
- Chapiro J, Claverol S, Piette F, et al. Destructive cleavage of antigenic peptides either by the immunoproteasome or by the standard proteasome results in differential antigen presentation. J Immunol 2006;176:1053-61. [Crossref] [PubMed]
- Tripathi SC, Peters HL, Taguchi A, et al. Immunoproteasome deficiency is a feature of non-small cell lung cancer with a mesenchymal phenotype and is associated with a poor outcome. Proc Natl Acad Sci U S A 2016. [Epub ahead of print]. [Crossref] [PubMed]
- Avalle L, Pensa S, Regis G, et al. STAT1 and STAT3 in tumorigenesis: a matter of balance. JAKSTAT 2012;1:65-72. [Crossref] [PubMed]
- Hugo W, Zaretsky JM, Sun L, et al. Genomic and transcriptomic features of response to anti-PD-1 therapy in metastatic melanoma. Cell 2016;165:35-44. [Crossref] [PubMed]
- Reits EA, Hodge JW, Herberts CA, et al. Radiation modulates the peptide repertoire, enhances MHC class I expression, and induces successful antitumor immunotherapy. J Exp Med 2006;203:1259-71. [Crossref] [PubMed]
- Verbrugge I, Gasparini A, Haynes NM, et al. The curative outcome of radioimmunotherapy in a mouse breast cancer model relies on mTOR signaling. Radiat Res 2014;182:219-29. [Crossref] [PubMed]