Quantitative analyzes of the variability in airways via four-dimensional dynamic ventilation CT in patients with chronic obstructive pulmonary disease: correlation with spirometry data and severity of airflow limitation
Highlight box
Key findings
• The dynamic coefficient of variance of minimum lumen diameter in RB1 could help to predict severe chronic obstructive pulmonary disease (COPD) patients via four-dimensional dynamic ventilation computed tomography (4D-CT).
What is known and what is new?
• COPD have complex pathologic abnormalities which are not fully evaluated by spirometry.
• The deformation of airways during the breathing cycle and the correlation of these dynamic changes with the severity of airflow limitation were explored.
What is the implication, and what should change now?
• 4D-CT might be an available method that can supplement the diagnosis of COPD and the evaluation of the severity of airflow limitation in COPD patients.
• More sample size need to conducted to verify the present results.
Introduction
The diagnosis of chronic obstructive pulmonary disease (COPD) and the evaluation of its airflow limitation severity are mainly based on the results of the pulmonary function test (PFT) (1). However, this test may lead to a misdiagnosis of COPD due to poor patient cooperation, particularly in elderly patients; moreover, acute exacerbation of COPD is a contraindication to the PFT. Furthermore, it has been shown that patients with COPD have complex pathologic abnormalities, including destruction of lung tissue (emphysema), airway inflammation and small airway impairment (2-4). Such abnormalities are not fully evaluable by spirometry alone (5), nor is the severity of the disease. Patients with severe COPD are often unwilling to cooperate with additional, laborious PFTs that may be unable to fully assess airway function. Hence, other methods are needed to supplement the diagnosis and assist in evaluating the severity of the condition in the absence of lung function data.
Advances in computed tomography (CT) technology has led to the development of thoracic four-dimensional dynamic ventilation CT (4D-CT), which combines temporal and spatial data to continuously capture the process of lung deformation during tidal breathing (6). This imaging technology complements lung morphology between respiratory phases, providing lung analysis of gas dynamics and pressure distribution that approximates true physiological function and visualizing continuous lung motion in disease states. Several studies have shown the high value of 4D-CT for diagnosing and evaluating other diseases, such as asthma (7,8). 4D-CT has advantages for evaluating COPD pathologies due to its ability to provide information on lower airway dynamics in a rapid and noninvasive manner, with little effort needed from patients. Studies have already demonstrated reduced synchrony between the proximal airway luminal area and mean lung density (MLD) associated with airway limitation, providing new insights into the pathological mechanism of COPD (9,10). In clinical practice, COPD patients with severe small airway obstruction exhibit decreased lung function and increased mortality (11,12). However, no study to date has explored the dynamic variability in 4th-generation airways in COPD patients; thus, accurate assessment of the airway geometry during the respiratory cycle with 4D-CT is needed. Given the increased small airway resistance in COPD patients, we hypothesized that 4D-CT, in addition to enabling visualization of airway movements, would provide new information for the quantitative assessment of airway dynamic variability; these data could be used to assess the severity of airflow limitation in COPD patients.
Thus, in the current study, we performed 4D-CT scans in COPD patients to assess the deformation of 4th-generation airways during the breathing cycle and to explore the correlation of these dynamic changes with the severity of airflow limitation. In addition, we hoped to establish an index to evaluate the dynamic changes in airways to compensate for poor patient cooperation and the inability of patients to hold their breath during conventional CT. We present this article in accordance with the STROBE reporting checklist (available at https://jtd.amegroups.com/article/view/10.21037/jtd-23-573/rc).
Methods
Subjects
Patients with COPD who presented to Ruijin Hospital between January 2021 and January 2022 were enrolled in the current study. These patients were diagnosed with COPD according to the criteria of the Global Initiative for Chronic Obstructive Lung Disease (GOLD) definition (1). COPD patients with other respiratory diseases, such as asthma, lung cancer, bronchiectasis, or interstitial lung diseases, were excluded. We collected demographic and clinical characteristics, including age, sex, body mass index (BMI), and smoking history. All subjects were further classified into two groups, namely, the GOLD I + II group (FEV1% ≥50%) and GOLD III + IV group (FEV1% <50%), according to their spirometry results for the purpose of analysis. The study was conducted in accordance with the Declaration of Helsinki (as revised in 2013). The study was approved by the Institutional Review Board of Shanghai Ruijin Hospital (No. 2020-105) and informed consent was taken from all the patients.
Dynamic-ventilation CT
All patients underwent 4D-CT. They were examined by a 320-row multidetector computed tomography (MDCT) scanner (Aquilion ONE; Canon Medical Systems Otawara, Tochigi, Japan) for dynamic ventilatory scans. Axial, dynamic scanning was performed with a coverage of 160 mm in the z-axis. Other scanning and reconstruction parameters for the dynamic ventilatory scan were as follows: tube currents =100 mA, tube voltage =80 kVp, rotation time =0.275 seconds; total scanning time =6.875 seconds (13 gantry rotations); imaging field of view (FOV) =400 mm, collimation =0.5 mm, slice thickness =0.5 mm, reconstruction kernel = FC09, with an iterative reconstruction method [adaptive iterative dose reduction using three-dimensional processing (AIDR3D)] (13). Before the dynamic ventilation CT scan, the patients were instructed to inhale and exhale to generate a consistent free breathing rhythm according to a spoken command. Thus, scans included at least one cycle (from inspiration to expiration).
Image analysis—quantitative airway measurement on dynamic ventilation CT
All scan data were anonymized. Using commercially available research software (4D Airways Analysis; Toshiba Medical Systems), continuous image data (320 images/frame × total 13 frames, every 0.55 seconds) were synthesized for the cine-CT series. Yamashiro et al. described the detailed information of the software in their technical note, including multi-level airway tree segmentation with internal feedback mechanism, centerline construction and optimization, synchronization for anatomical location and context constrained wall contour estimation (14). In brief, the software automatically created a tree of the centre lines from the trachea to all traceable peripheral bronchi throughout the cine-CT (Figure 1A), and a measurement line (orange line) was set at the midpoint (the midpoint between the parent and the daughter branch points, as manually marked by the radiologist) of the bronchus. To ensure the consistency of measurement and comparability of results, parameters were measured in the 4th-generation airways in RB1 (apical segment of the upper lobe in the right lung), RB4 (lateral segment of the middle lobe in the right lung), and RB10 (posterior basal segment of the lower lobe in the right lung) (Figure 1A). Then, the minimum lumen diameter in all 13 time frames during free breathing was measured automatically (Figure 1B,1C).
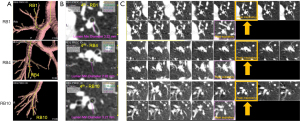
In this study, the minimum airway diameter values were defined as the minimum diameter of the inner lumen. On the time curve, the minimum lumen diameter values are plotted in each of 13 frames (0.55 seconds/frame), and then the minimum lumen diameter in the peak inspiratory frame and the peak expiratory frame as well as the peak expiratory/peak inspiratory ratio (E/I ratio), and the coefficient of variance (CV) of the minimum airway diameter values [% = 100 × standard deviation (SD)/mean], were calculated.
The E/I ratio of the minimum lumen diameter was used to quantitatively calculate the collapsibility of the airways. The CV is essentially the SD normalized to the mean minimum lumen diameter during the 13 time frames; this value reflects the dynamic variability in 4th-generation airway dimensions during respiration (Figure 2). Similar to a previous analysis, we also calculated average values of minimum lumen diameter in 4th-generation bronchi of the RB1, RB4 and RB10 bronchial segments (15).

Spirometry
All subjects underwent spirometry according to American Thoracic Society standards (16), including the following measurements and calculations: forced expiratory volume in 1 second (FEV1), forced expiratory volume in one second/forced vital capacity (FEV1/FVC), forced expiratory volume in three seconds/forced vital capacity (FEV3/FVC), maximal expiratory flow 75% (MEF75), maximal expiratory flow 50% (MEF50), maximal expiratory flow 25% (MEF25), and maximal mid-expiratory flow (MMEF). Spirometry was performed within 1 month of the four-dimensional CT.
Statistical analysis
All statistical analyzes were performed using SPSS version 26.0 statistical software (IBM Corporation, Armonk, NY, USA). All quantitative parameters are expressed as the means ± standard deviations (SDs) or median and interquartile (25th–75th percentiles), as appropriate. The Kolmogorov-Smirnov tests were performed to test for a normal distribution. Differences in clinical characteristics between the two groups were evaluated using t-tests and Mann-Whitney U tests for continuous variables, and the chi-squared test and Fisher’s exact probability method were used for categorical variables. Spearman’s correlation analysis was used to evaluate the correlation between the CT indexes and the spirometric values. Furthermore, receiver operating characteristic (ROC) curves and the area under the curve (AUC) were employed to evaluate the ability of the CV of minimum lumen diameter in RB1 to predict FEV1% <50%, as well as its cut-off value. All statistical tests were two-sided, and a P value <0.05 was considered significant.
Results
Clinical characteristics
Forty-two patients were included in the study {forty-one males and one female; median age: 68 years [interquartile range (IQR), 61–73 years]}. Based on their FEV1%, 21 patients (50.00%) were categorized as GOLD I + II, and 21 patients (50.00%) were categorized as GOLD III + IV. Table 1 summarizes the characteristics of the enrolled patients, including their spirometric values. The median BMI was 22.73 kg/m2 (IQR, 20.66–25.11 kg/m2), and the median pack-years smoked value was 40.00 (IQR, 29.25–53.19). The patients showed airflow limitation, with a low predicted FEV1% [49.95% (IQR, 39.65–67.25%)] and poor small airway function indexes of MEF75 [1.66 L/s (IQR, 1.08–3.12 L/s)], MEF50 [0.74 L/s (IQR, 0.44–1.53 L/s)], MEF25 [0.29 L/s (IQR, 0.20–0.42 L/s)] and MMEF [0.61 L/s (IQR, 0.39–1.08 L/s)].
Table 1
Characteristics | Total |
---|---|
N | 42 |
Age, years | 68.00 (61.00–73.00) |
Male, n (%) | 41 (97.6) |
BMI, kg/m2 | 22.73 (20.66–25.11) |
Pack-years smoked | 40.00 (29.25–53.19) |
GOLD I + II/III + IV | 21/21 |
Predicted FEV1, % | 49.95 (39.65–67.25) |
FEV1/FVC, % | 55.88 (45.28–68.18) |
FEV3/FVC, % | 81.65 (72.58–89.29) |
MEF75 (L/s) | 1.66 (1.08–3.12) |
MEF50 (L/s) | 0.74 (0.44–1.53) |
MEF25 (L/s) | 0.29 (0.20–0.42) |
MMEF (L/s) | 0.61 (0.39–1.08) |
Data are presented as median (interquartile 25th–75th percentiles) unless specified otherwise. BMI, body mass index; GOLD, Global Initiative For Chronic Obstructive Lung Disease; FEV1, forced expiratory volume in one second; FEV1/FVC, forced expiratory volume in 1 second/forced vital capacity; FEV3/FVC, forced expiratory volume in three second/forced vital capacity; MEF75, maximal expiratory flow 75%; MEF50, maximal expiratory flow 50%; MEF25, maximal expiratory flow 25%; MMEF, maximal mid-expiratory flow.
Dynamic changes in the minimum lumen diameter of 4th-generation airways
4D-CT provided continuous measures of airway motion during respiration and clearly showed airway distention and collapse. Airway dimensions of different 4th-generation bronchi, including the peak inspiratory frame, peak expiratory frame, E/I ratio and CV, were calculated to determine the dynamic changes in airways of COPD patients. The median (IQR) minimum lumen diameter in the peak inspiratory frame of RB1, RB4, and RB10 bronchi segments was 2.26 (1.92–2.53), 1.92 (1.43–2.41), and 2.90 (2.42–3.39) mm, respectively. The minimum airway diameter changed during the respiratory cycle, gradually decreasing during exhalations. The median (IQR) minimum lumen diameter in the peak expiratory frame of RB1, RB4, and RB10 bronchi segments was 1.59 (1.18–2.07), 1.37 (1.15–1.89), and 1.70 (1.32–2.28) mm, respectively. The collapsibility of the airways in terms of the median (IQR) of the E/I ratio in the RB1, RB4, and RB10 bronchi segments was 0.79 (0.62–0.87), 0.78 (0.61–0.93), 0.59 (0.50–0.70) mm, respectively. The dynamic CV of minimum lumen diameter in RB1, RB4, and RB10 bronchi segments was 12.03% (7.98–21.82%), 16.50% (12.10–23.14%), 21.86% (16.03–28.36%), respectively. Moreover, the values of the RB1, RB4 and RB10 segments were also averaged to reflect the dynamic parameters of the whole right lung, as shown in Table 2.
Table 2
Variable | Inspiration (mm) | Expiration (mm) | E/I ratio | Coefficient of variance, % |
---|---|---|---|---|
RB1 | 2.26 (1.92–2.53) | 1.59 (1.18–2.07) | 0.79 (0.62–0.87) | 12.03 (7.98–21.82) |
RB4 | 1.92 (1.43–2.41) | 1.37 (1.15–1.89) | 0.78 (0.61–0.93) | 16.50 (12.10–23.14) |
RB10 | 2.90 (2.42–3.39) | 1.70 (1.32–2.28) | 0.59 (0.50–0.70) | 21.86 (16.03–28.36) |
Average | 2.30 (2.03–2.57) | 1.56 (1.32–1.94) | 0.71 (0.66–0.82) | 15.94 (11.98–23.99) |
Data are presented as median (interquartile 25th–75th percentiles). The independent bronchi and average values of minimum lumen diameter in 4th-generation bronchi were from the RB1, RB4 and RB10 bronchial segments. E/I, expiratory/inspiratory; coefficient of variance, % = 100 × standard deviation/mean; RB1, apical segment of the upper lobe in the right lung; RB4, lateral segment of the middle lobe in the right lung; RB10, posterior basal segmental of the lower lobe in the right lung.
Analyzes of the correlation between 4D-CT values and spirometric values
Figure 3 shows Spearman’s correlation coefficient of all patients with different bronchi segments. We found a stronger overall correlation of spirometric values with the CV than with the other three parameters. Correlations of FEV1% with the CV of RB1 (ρ=−0.473, P=0.002), RB10 (ρ=−0.480, P=0.005) and average (ρ=−0.495, P=0.001) were observed. Additionally, correlations of FEV1/FVC with the CV of RB1 (ρ=−0.487, P=0.001), RB10 (ρ=−0.372, P=0.033) and average (ρ=−0.502, P=0.001) were noted. We also found correlations of FEV3/FVC with the CV of RB1 (ρ=−0.521, P<0.001), RB10 (ρ=−0.444, P=0.011) and average (ρ=−0.549, P<0.001). Next, we further analyzed the correlation between the CV and small airway function indexes, including MEF75, MEF50, MEF25 and MMEF. The highest Spearman’s correlation coefficients were between MEF75 and CV-Average (ρ=−0.558, P<0.001), MEF50 and CV-Average (ρ=−0.583, P<0.001), MEF25 and CV-RB10 (ρ=−0.555, P=0.001), and MMEF and CV-Average (ρ=−0.574, P<0.001), respectively. These findings indicate that the dynamic variability in 4th-generation airway caliber was associated with airflow limitation and small airway dysfunction in COPD patients.
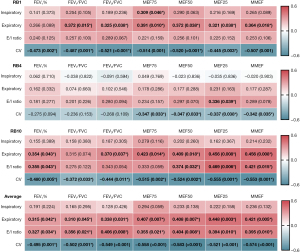
Group differences in the 4D-CT indexes of 4th-generation airways
Based on their FEV1%, 21 patients (50.00%) were assigned to the GOLD I + II group, and 21 patients (50.00%) were assigned to the GOLD III + IV group. Airway caliber in different phases of the respiratory cycle, including the peak inspiratory frame and peak expiratory frame as well as the dynamic obstruction severity rate, including the E/I ratio and CV, were measured to understand the morphological changes in 4th-generation airways with the progression of COPD (Figure 4 and Table S1). CV-RB1 significantly differed between the GOLD I + II and GOLD III + IV groups [8.59 (IQR, 6.63–14.86) vs. 14.64 (10.65–25.88), respectively; P=0.016]. In contrast, CV-RB4 [15.62 (IQR, 12.51–21.88) vs. 17.24 (IQR, 11.80–23.81); P=0.897], CV-RB10 [18.28 (IQR, 15.62–25.89) vs. 23.44 (IQR, 16.31–28.89); P=0.331] and CV-Average [15.31 (IQR, 10.73–21.30) vs. 18.72 (IQR, 13.24–25.54); P=0.116] were not significantly different between the two groups. These data indicate that the GOLD III + IV group exhibited increased airways dynamic variability.
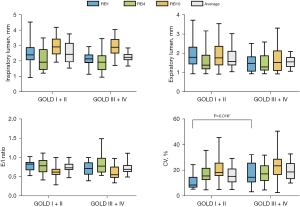
CV-RB1 predicted FEV1% <50% in COPD patients
As CV-RB1 was higher in the GOLD III + IV group, which included patients with more severe airflow limitations than the GOLD I + II group, this value could be used as a sensitive marker for predicting FEV1% <50% in cases with missing spirometry data. To better analyze the predictive ability of CV-RB1, we used “FEV1% <50%” as the outcome in the predictive model. Based on the ROC curve analysis (Figure 5A), the AUC for CV-RB1 was 0.717 (95% CI: 0.560–0.873; P=0.016). With an optimal cut-off of 9.43%, the sensitivity and specificity of CV-RB1 for predicting FEV1% <50% were 85.7% and 57.1%, respectively. Figure 5B shows the linear regression analysis of the association between CV-RB1 and FEV1%.

Discussion
The current study analyze the dynamic variability in the dimensions of 4th-generation airways in COPD patients. Our main findings were as follows: (I) 4D-CT quantitatively reflects the dynamic changes in airways in patients with COPD during free breathing; (II) the dynamic coefficient of variance (CV) of 4th-generation airway diameter was significantly correlated with PFT test values; and (III) CV of minimum lumen diameter in RB1 can be used to predict COPD patients with FEV1% <50% in the absence of spirometry data. 4D-CT enables visualization of airway changes and flexibly reflects the collapse of the airways during the breathing cycle, without limitations to a single phase as in the data from static CT. Thus, we suggest that 4D-CT complements existing methods for diagnosis of COPD and identification of patients with severe airflow limitation.
4D-CT provides continuous numeric measures of airway lumen diameter, making it easier to perform statistical comparisons of dynamic parameters. The tracheal tree and time course of the tracheal morphology segmented by 4D-CT allows visualization of the trachea expanding during inspiration and contracting during expiration, providing a comprehensive assessment of lung and airway motion. The quantitative data generated by 4D-CT can be used to assess the dynamic parameters of the trachea, in addition to that of small airways, which are essential for disease progression in COPD. Previous studies have used 4D-CT to explore large airways (9,10,17); however, research has overlooked the dynamic assessment of small airways. Small airways (<2 mm in diameter; 4th–12th-generation airways) are the primary pathological site of airflow limitation in COPD (18,19). Small airway obstruction is an important part of the progression of COPD, and airway narrowing is one of the pathological features of COPD (20,21).
Before being diagnosed with COPD, patients may have presented with small airway dysfunction, including narrowing and reduction in the number of small airways. As the disease progresses, the decline in lung function becomes more pronounced, and the small airway lesions become more severe (11). Therefore, the dynamic CV of airway diameter, as calculated quantitatively by 4D-CT here, accurately reflects this drastic change in the respiratory cycle and helps to explore the correlation between the dynamic instability of airways and airflow obstruction.
We found a higher overall correlation coefficient between the CV and spirometric values. This finding indicates that the dynamic variability in 4th-generation airway caliber was associated with airflow limitation and small airway dysfunction in COPD patients. In patients with severe COPD with distal airway stenosis, lumen diameter stenosis causes an exponential increase in airflow resistance (22). In this condition, the airflow output is difficult, and the respiratory flow rate is decreased, leading to airway collapse during expiration and instability of small airways. These features may explain the exaggerated narrowing of the airway diameter observed in 4D-CT, which can lead to air trapping and clinical manifestations such as decreased exercise tolerance.
A previous study analyzed airway cross-sectional measurements, including inspiratory luminal area, expiratory luminal area, and the E/I ratio; these measurements were not significantly correlated with FEV1/FVC (9). The end-inspiratory and end-expiratory phases are usually selected to measure airway dimensions such as the luminal diameter and airway wall percent (23,24). Parametric Response Mapping (PRM) was selected to provide a classification of lung parenchyma as normal, functional small airways disease and emphysema (25). However, 4D-CT provides further data regarding changes over time. Interestingly, we found that in a respiratory cycle, airway movement underwent a complex physiological change. Thus, we selected the minimum lumen diameter in the peak inspiratory frame and peak expiratory frame as well as the E/I ratio and dynamic CV, which cannot be calculated from one scan of conventional CT data. Intriguingly, we found that the dynamic CV of 4th-generation airways was negatively correlated with FEV1%, FEV1/FVC, FEV3/FVC, MEF75, MEF50, MEF25 and MMEF in COPD patients; these negative correlations were stronger than the correlation between the other three parameters (i.e., the E/I ratio and minimum lumen diameter in the peak inspiratory and expiratory frames) and spirometric values. This phenomenon may be attributable to a large decrease in small airway dimensions during expiration, which is associated with airway obstruction. O’Donnell et al. observed a wide range of end-expiratory tracheal collapse among patients with COPD, with most of the change in area resulting from a reduction in sagittal diameter (26). We speculate that occlusion of peripheral airways may result in airway limitation. This occlusion may be a target for new management strategies to improve symptom relief. Nevertheless, the CV in RB4 showed a weak correlation with the PFT values. One possible reason is that the middle lobe of the lung has a horizontal alignment, which is not conducive to segmentation of the airway by the analysis software; thus, its cross-section may not provide a good estimation of the true dimensions of the lumen compared to the vertical alignment of the airways in the upper and lower lobes of the lung.
We also divided all patients into two groups based on the severity of airflow limitation, namely, the GOLD I+II and GOLD III + IV groups. In the present study, 4D-CT technology was employed to explain the mechanism underlying group differences. We found that the dynamic CV of minimum lumen diameter in RB1 was significantly higher in the GOLD III+IV group and that the pulmonary ventilation function of this group was worse. 4D-CT is also recommended to evaluate airway stability in patients with severe COPD (17). For example, Yamashiro et al. found that a heterogenous distribution of pulmonary emphysema might impact the synchrony of respiratory movements between the lung lobes in patients with severe COPD (27). As there was a significant correlation between the dynamic change rate of 4th-generation airways and spirometric values, 4D-CT is a good choice for patients who have difficulty cooperating with the PFT or are unable to hold their breath during conventional chest CT. We demonstrated that a CV of minimum lumen diameter in RB1 greater than 9.43% is a sensitive marker for FEV1% <50%. This is the first study to use 4D-CT to describe 4th-generation airway movements during the actual breathing process as well as differences between patients with different severities of diseases. Continuous measurements revealed that the effect of cardiac motion on the apex of the right upper lung lobe was almost negligible. Previous studies have shown a high agreement between the right upper lobe and the whole lung (28); additionally, in male smokers, the airway wall percentage in RB1 is representative of the whole lung (29).
COPD is a very heterogeneous disease involving airflow limitation and can be caused by increased small airway resistance and/or terminal airspace emphysematous changes. Conventional CT usually requires breath-holding; however, in patients with severe COPD, breath-holding is often difficult and there is a risk of bullae rupture; thus, continuous image acquisition by 4D-CT has unique advantages in terms of eliminating breathing artefacts and reducing patient effort. Information for evaluating airways’ dynamic CV may help improve treatment strategies.
Our study demonstrates the feasibility of 4D-CT as a new imaging tool to accurately assess airway respiratory dynamics, especially when applied to the assessment of lumen stability in patients with severe COPD. This method can provide valuable clinical information for diagnostic and interventional treatment. Future studies need to analyze a larger number of subjects to determine differences in characteristics between COPD patients and healthy populations; additionally, advances in CT technology may increase resolution while decreasing the radiation dose. Given the limited sample size, in this study, we focused on analyzing the correlation between dynamic variability in 4th-generation airways and airflow obstruction, such as FEV1% and MMEF, in COPD patients. We compared patients with different severities of COPD and predicted those with FEV1% <50% according to heterogeneous and dynamic indicators. These data may help in clinical practice to assess the severity of airflow obstruction and provide a powerful imaging tool for patients who are unable to cooperate with the PFT and conventional CT.
This study had several limitations. First, as the application of 4D-CT technology in the COPD population is still in the exploratory stage, the total number of enrolled patients was small. Second, only 4th-generation airways in the right lung were investigated in this study due to the rapid increase in the number of 4D-CT images. Third, paradoxical movement of airway diameters in severe COPD patients may disturb the measurement in peak inspiratory and peak expiratory. Further research is needed to explore the movement of peripheral small airways and fully evaluate the dynamic changes of all segments in the whole lung.
Conclusions
4D-CT revealed that the dynamic variability in 4th-generation airway caliber was correlated with spirometric values in patients with COPD. The CV of 4th-generation airway variability was significantly increased in the GOLD III + IV group. 4D-CT might be an available method (in addition to the PFT) that can supplement the diagnosis of COPD and the evaluation of the severity of airflow limitation in COPD patients.
Acknowledgments
The authors thank Jing Yan (Canon Medical System, Shanghai, China) and Qun Han (Department of CT Advanced Clinical Application, Philips Healthcare) for their great technical support.
Funding: This work was supported by
Footnote
Reporting Checklist: The authors have completed the STROBE reporting checklist. Available at https://jtd.amegroups.com/article/view/10.21037/jtd-23-573/rc
Data Sharing Statement: Available at https://jtd.amegroups.com/article/view/10.21037/jtd-23-573/dss
Peer Review File: Available at https://jtd.amegroups.com/article/view/10.21037/jtd-23-573/prf
Conflicts of Interest: All authors have completed the ICMJE uniform disclosure form (available at https://jtd.amegroups.com/article/view/10.21037/jtd-23-573/coif). The authors have no conflicts of interest to declare.
Ethical Statement:
Open Access Statement: This is an Open Access article distributed in accordance with the Creative Commons Attribution-NonCommercial-NoDerivs 4.0 International License (CC BY-NC-ND 4.0), which permits the non-commercial replication and distribution of the article with the strict proviso that no changes or edits are made and the original work is properly cited (including links to both the formal publication through the relevant DOI and the license). See: https://creativecommons.org/licenses/by-nc-nd/4.0/.
References
- Global Initiative for Chronic Obstructive Lung Disease (GOLD). Global Strategy for the Diagnosis, Management, and Prevention of Chronic Obstructive Pulmonary Disease (2021 REPORT). Available online: http://www.goldcopd.org
- Ju J, Li R, Gu S, et al. Impact of emphysema heterogeneity on pulmonary function. PLoS One 2014;9:e113320. [Crossref] [PubMed]
- Bodduluri S, Kizhakke Puliyakote A, Nakhmani A, et al. Computed Tomography-based Airway Surface Area-to-Volume Ratio for Phenotyping Airway Remodeling in Chronic Obstructive Pulmonary Disease. Am J Respir Crit Care Med 2021;203:185-91. [Crossref] [PubMed]
- Liu H, Li J, Ma Q, et al. Chronic exposure to diesel exhaust may cause small airway wall thickening without lumen narrowing: a quantitative computerized tomography study in Chinese diesel engine testers. Part Fibre Toxicol 2021;18:14. [Crossref] [PubMed]
- Andreeva E, Pokhaznikova M, Lebedev A, et al. Spirometry is not enough to diagnose COPD in epidemiological studies: a follow-up study. NPJ Prim Care Respir Med 2017;27:62. [Crossref] [PubMed]
- Jahani N, Choi S, Choi J, et al. Assessment of regional ventilation and deformation using 4D-CT imaging for healthy human lungs during tidal breathing. J Appl Physiol (1985) 2015;119:1064-74. [Crossref] [PubMed]
- Jahani N, Choi S, Choi J, et al. A four-dimensional computed tomography comparison of healthy and asthmatic human lungs. J Biomech 2017;56:102-10. [Crossref] [PubMed]
- Ueha R, Maeda E, Ino K, et al. Sleep-Induced Glottis Closure in Multiple System Atrophy Evaluated by Four-Dimensional Computed Tomography. Front Med (Lausanne) 2020;7:132. [Crossref] [PubMed]
- Yamashiro T, Moriya H, Tsubakimoto M, et al. Continuous quantitative measurement of the proximal airway dimensions and lung density on four-dimensional dynamic-ventilation CT in smokers. Int J Chron Obstruct Pulmon Dis 2016;11:755-64. [Crossref] [PubMed]
- Nagatani Y, Hashimoto M, Nitta N, et al. Continuous quantitative measurement of the main bronchial dimensions and lung density in the lateral position by four-dimensional dynamic-ventilation CT in smokers and COPD patients. Int J Chron Obstruct Pulmon Dis 2018;13:3845-56. [Crossref] [PubMed]
- McDonough JE, Yuan R, Suzuki M, et al. Small-airway obstruction and emphysema in chronic obstructive pulmonary disease. N Engl J Med 2011;365:1567-75. [Crossref] [PubMed]
- Hogg JC, Chu FS, Tan WC, et al. Survival after lung volume reduction in chronic obstructive pulmonary disease: insights from small airway pathology. Am J Respir Crit Care Med 2007;176:454-9. [Crossref] [PubMed]
- Yamashiro T, Miyara T, Honda O, et al. Adaptive Iterative Dose Reduction Using Three Dimensional Processing (AIDR3D) improves chest CT image quality and reduces radiation exposure. PLoS One 2014;9:e105735. [Crossref] [PubMed]
- Yamashiro T, Tsubakimoto M, Nagatani Y, et al. Automated continuous quantitative measurement of proximal airways on dynamic ventilation CT: initial experience using an ex vivo porcine lung phantom. Int J Chron Obstruct Pulmon Dis 2015;10:2045-54. [Crossref] [PubMed]
- Nambu A, Zach J, Schroeder J, et al. Quantitative computed tomography measurements to evaluate airway disease in chronic obstructive pulmonary disease: Relationship to physiological measurements, clinical index and visual assessment of airway disease. Eur J Radiol 2016;85:2144-51. [Crossref] [PubMed]
- Vestbo J, Hurd SS, Agustí AG, et al. Global strategy for the diagnosis, management, and prevention of chronic obstructive pulmonary disease: GOLD executive summary. Am J Respir Crit Care Med 2013;187:347-65. [Crossref] [PubMed]
- Wielpütz MO, Eberhardt R, Puderbach M, et al. Simultaneous assessment of airway instability and respiratory dynamics with low-dose 4D-CT in chronic obstructive pulmonary disease: a technical note. Respiration 2014;87:294-300. [Crossref] [PubMed]
- Hogg JC, Macklem PT, Thurlbeck WM. Site and nature of airway obstruction in chronic obstructive lung disease. N Engl J Med 1968;278:1355-60. [Crossref] [PubMed]
- Hogg JC, Chu F, Utokaparch S, et al. The nature of small-airway obstruction in chronic obstructive pulmonary disease. N Engl J Med 2004;350:2645-53. [Crossref] [PubMed]
- Singh D. Small Airway Disease in Patients with Chronic Obstructive Pulmonary Disease. Tuberc Respir Dis (Seoul) 2017;80:317-24. [Crossref] [PubMed]
- Brashier BB, Kodgule R. Risk factors and pathophysiology of chronic obstructive pulmonary disease (COPD). J Assoc Physicians India 2012;60:17-21. [PubMed]
- McNulty W, Usmani OS. Techniques of assessing small airways dysfunction. Eur Clin Respir J 2014; [Crossref] [PubMed]
- Diaz AA, Come CE, Ross JC, et al. Association between airway caliber changes with lung inflation and emphysema assessed by volumetric CT scan in subjects with COPD. Chest 2012;141:736-44. [Crossref] [PubMed]
- Yamashiro T, Matsuoka S, Estépar RS, et al. Quantitative assessment of bronchial wall attenuation with thin-section CT: An indicator of airflow limitation in chronic obstructive pulmonary disease. AJR Am J Roentgenol 2010;195:363-9. [Crossref] [PubMed]
- Konietzke P, Wielpütz MO, Wagner WL, et al. Quantitative CT detects progression in COPD patients with severe emphysema in a 3-month interval. Eur Radiol 2020;30:2502-12. [Crossref] [PubMed]
- O'Donnell CR, Bankier AA, O'Donnell DH, et al. Static end-expiratory and dynamic forced expiratory tracheal collapse in COPD. Clin Radiol 2014;69:357-62. [Crossref] [PubMed]
- Yamashiro T, Moriya H, Matsuoka S, et al. Asynchrony in respiratory movements between the pulmonary lobes in patients with COPD: continuous measurement of lung density by 4-dimensional dynamic-ventilation CT. Int J Chron Obstruct Pulmon Dis 2017;12:2101-9. [Crossref] [PubMed]
- Tho NV. Airway wall area derived from 3-dimensional computed tomography analysis differs among lung lobes in male smokers. PLoS One 2014;9:e98335. [Crossref] [PubMed]
- Nakano Y, Muro S, Sakai H, et al. Computed tomographic measurements of airway dimensions and emphysema in smokers. Correlation with lung function. Am J Respir Crit Care Med 2000;162:1102-8. [Crossref] [PubMed]