Association between NADPH oxidase (NOX) and lung cancer: a systematic review and meta-analysis
Introduction
Oxidation/reduction imbalance occurs in the milieu of chronic inflammation in the tissues and may contribute to the pathogenesis of a variety of human cancers (1,2). Oxidative stress-induced tumor initiation or progression may result from overproduction of reactive oxygen species (ROS) by members of the NADPH oxidase (NOX) family (3).
The family of NOXs is consisted of seven members, that is, NOX1–5 and dual oxidases (DUOX)-1 and DUOX-2. NOXs are structurally homologues of gp91phox, the major membrane-bound component of the respiratory burst oxidase (4). In mammals, production of H2O2 is predominantly mediated by NOX 4 and DUOX-1 and -2, while the remaining four family members (NOX 1–3, and NOX 5) produce superoxide (4,5). Each NOX/DUOX protein is anchored to the plasma membrane through six transmembrane helices, which bind two heme cofactors. A C-terminal FAD-/NADPH-binding domain facilitates electron transfer to the heme molecules for ROS production. In addition, DUOX-1 and -2 possess extracellular peroxidase-like domains that are responsible for their descriptive nomenclature, and their functions remain an area of major research interest (6,7).
Originally, it was thought that NOXs existed only in phagocytic cells to protect the host against pathogenic organisms. However, over the last 15 years, homologues of NOXs have been discovered in a variety of different cell and tissue types including cancer cells, and the isoform expression is restricted by tissue and subcellular context (8). In this content, lung cancer tissue or cell lines predominantly express NOX 4 isoform (9,10). In addition, NOX 4 is expressed in distal tubular cells of the cortex of the kidney and in endothelial cells. NOX 4 expression has also been found in other tumors such as ovary and brain tumor (11,12). DUOX-1 and -2 are prominent in airway epithelial cells, where it plays a critical role in host defense (13). However, it has been reported that mRNA of DUOX-1 and -2 are reduced in lung cancer samples compared with adjacent normal lung tissues, which results from site-specific methylation of the DUOX promoter (14).
For optimal function and activity, NOXs associate with membrane-bound p22phox and soluble subunit analogues of both p47phox and p67phox, as well as the small GTPase Rac1 for efficient electron transport across the membrane (15,16). Recently, knowledge of the roles of the NOX isoforms in cancer development and metastasis is rapidly expanding. In this regard, accumulating evidence suggests that both NOX 1 and DUOX-2 may contribute to the development of colorectal and pancreatic carcinomas in patients with inflammatory bowel disease (17,18), and that NOX 4 expression is increased in pre-malignant fibrotic states which may lead to carcinomas of the lung (19).
While the mechanism of NOX-mediated carcinogenesis remains to be defined, over-expression of functional NOX proteins leading to tissue injury and DNA damage from ROS explains, which, at least in part, leads to pre-malignant conditions in many tissues and may contribute to both the initiation and the progression of a wide range of malignancies including lung cancer. In this context, recent studies suggest that NOXs activity or mRNA expression is up-regulated in lung cancer, and that ROS production by NOX species can strongly influence both tumor growth and survival of lung cancer cells (20,21). Furthermore, inhibition of NOX activity or mRNA expression resulted in suppression of lung cancer cell invasion or migration (22-24). On the other hand, studies also fund that DUOX-1 and DUOX-2 mRNA expression was significantly decreased in lung cancer compared to its adjacent normal tissue (14). These publications indicate that NOXs may contribute to the development of lung cancer. Therefore, we have systematically reviewed publications and performed meta-analysis to examine association between NOXs expression or functional activity and in vitro lung cancer cell invasion or in vivo lung cancer nodule formation.
Methods
Data sources
This systematic review and meta-analysis followed the Preferred Reporting Items for Systematic Reviews and Meta-analyses (PRISMA) criteria (25). Relevant literature was searched in the sites of PubMed, Embase and Web of Science with the following phrases: “NADPH oxidases” AND “lung cancer”, or “NOX” AND “lung cancer”. The search was limited to English, and relevant studies were also identified by hand-searching the references of included articles. Literature search was performed by the following authors: Ming Han, Tianhui Zhang, Lei Yang, and Zitong Wang.
Inclusion criteria
Studies were included in the current systematic review and meta-analysis if: (I) studies on the relationship between NOXs including DUOXs and lung cancer in patients or animals; (II) studies on the expression of mRNA or protein of NOXs and DUOXs in lung cancer tissues or cells; (III) studies on the activity of NOXs and DUOXs in lung cancer tissues or cells; (IV) studies with full text articles so that raw data could be extracted for the meta-analysis.
Data extraction
Data extraction was carried out by the following authors: Ming Han, Tianhui Zhang, and Lei Yang. Information and data were carefully extracted from all included literature according to the inclusion criteria as aforementioned. Data include study name (the first author name), publication date, study design, total number of cases or replication of the experiment, isoforms of NOXs that was investigated in the study, and inhibitors of NOX used in the study.
Statistical analysis
The following forms of data were used for the data entry: (I) mean, standard deviation (SD), number of cases or specimens; (II) sample size of lung cancer, sample size of control, and P value of comparison between the two groups; (III) event number in treated with NOX inhibitor(s) or siRNA, total number of the treated, event number of the non-treated, total number of the non-treated. The strength of association between NOX level and effect of NOX inhibition on lung cancer was measured by Hedges’s g; level of NOX expression or activity in lung cancer tissues or cell lines versus normal was measured by Hedges’s g; and the contribution of NOX level to lung cancer cell invasion or migration ability was measured by rate ratio. A fixed effect model was adopted when no heterogeneity was observed among the studies. Otherwise, a random effect model was applied. The heterogeneity between studies was assessed by the Q-test and I2 statistic, and P<0.10 and I2>50% was considered as heterogeneous between the studies (26). All meta-analysis was performed using the Comprehensive Meta-analysis software (Version 3, NJ, USA).
Results
Study features
The process of selecting literature was outlined as in Figure 1. After careful reading “abstract” of publications, total 77 full-text articles were retrieved. The retrieved full-text articles were then independently assessed by two investigators (MH, TZ). Ten articles were finally included in the systematic review, as shown in Table 1, including studies of the level of NOXs including DUOX1/2 activity or expression in human lung cancer tissue compared to normal tissue (n=4) (9,14,27,28), studies on the association of NOX level and occurrence of metastatic lung cancer (n=3) (9,29,30), and studies on correlation of NOX level and lung cancer cell invasion or migration ability (n=4) (10,22-24). Among the 10 articles, 4 articles were from USA, 4 from China, one from Italy and one from Finland. While six studies were performed using human lung tissue of cancer and its adjacent normal lung tissue and two were conducted in animals, none of these studies were clinical trials or performed in lung cancer patients. Publication bias was examined by plotting a funnel plot (Figure S1) that distributed nearly in symmetry, indicating publication bias not existed in the current study.
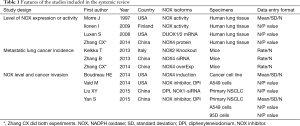
Full table
Results of overall analysis and subgroup analysis
When overall effect size of NOX/DUOX level and its effect on lung cancer cell function in seven studies were pooled into the meta-analysis, random effect model was used in that there was heterogeneity among the studies (I2=86, P<0.01). As shown in Figure 2, there was statistically significant effect of NOX/DUOX level or activity on lung cancer cell invasion or migration, effect size (Hedges’s g) =1.216, 95% confidence interval (95% CI): 0.089–2.343, and P=0.034.
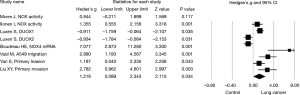
Next, effect size of NOX expression or activity and NOX inhibitor on lung cancer cell invasion or migration was assessed in subgroups. Again, a random effect model was adopted in assessing the effect size of NOX/DUOX expression or activity in lung cancer tissue or cells due to the high heterogeneity (I2=87.9, P<0.01). Effect size of lung cancer on NOX activity (2 studies) or mRNA expression level (DUOX-1, DUOX-2 or NOX 4) was not statistically significant (Hedges’s g =0.610, P=0.384, Figure 3). Effect size of NOX inhibition by molecular strategy (siRNA) or pharmacological inhibitors on primary lung cancer cell or cell line invasion, however, was statistically significant in favor of NOX inhibition (Hedges’s g =2.422, P<0.001, Figure 4). Furthermore, a fixed effect model was used in assessing the effect of NOX inhibition, which was conducted in three studies with seven different conditions, in that no heterogeneity was observed in this subgroup (I2=22.03, P=0.261).
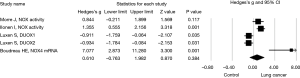
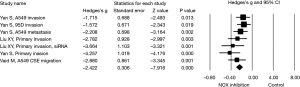
Last, effect size of NOX level in cancer cells (two studies) or animals (one study) on in vivo tumor formation or metastasis to the lung was evaluated. Animals lack of NOX 2 (29) had fewer number of Lewis lung carcinoma (LLC) compared to wild animals; and suppression of NOX 4 in cancer cell lines resulted in fewer number of tumor formation or metastasis in the animals when the cancer cells were injected into the animals (9,30). The effect size of NOX inhibition on lung cancer formation was statistically significant by a random effect model (rate ratio =0.366 with 95% CI: 0.196–0.684, P=0.002, Figure 5) with significant heterogeneity (I2=99, P<0.001).
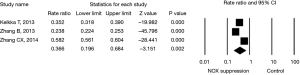
Discussion
Lung cancer is a worldwide health problem with leading cause of death due to its high incidence. More than 80% of lung cancers are non-small cell lung cancer (NSCLC). While genetic, epigenetic and environmental factors contribute to the tumorigenesis, chronic inflammation and NOX-derived ROS are also believed to play an important pathogenic role for several pre-malignant or malignant disease processes including lung cancer development. Therefore, in the current systematic review and meta-analysis, a potential role of NOX isoforms including DUOX in tumorigenesis of lung cancer was explored. To accomplish this, literature was searched in electronic databases including Embase, PubMed, and Web Science, using the following terms: “NADPH oxidases” and “Lung cancer”; “NOXs” and “Lung cancer”. Total 77 full-text publications were retrieved, and ten studies were included in the current review after careful screening. Overall meta-analysis of NOX/DUOX level in lung cancer tissue or cells and its effect on lung cancer cell invasion revealed that the pooled effect size of NOX/DUOX on lung cancer cell invasion and migration was statistically significant. In addition, the pooled effect size of NOX/DUOX inhibition by a pharmacologic inhibitor or siRNA on cancer cell invasion or migration was also statistically significant. These findings suggest that NOX/DUOX may be associated with tumorigenesis of lung cancer and could be a therapeutic target of lung cancer.
In vitro culture model system has been used to study the role of NOX- or DUOX-dependent ROS production in tumor cell growth, adhesion and invasion (22,24,31). In this context, it has been reported that over 100 human tumor cell lines express all the NOX isoforms and related genes (31,32). Specifically, NOX 1 expression at high levels was observed in H226 non-small cell lung cancer; NOX 2 was predominantly expressed in hematopoietic malignancies; robust NOX 4 expression was found in lung cancer, melanoma and ovarian cancer cell lines; NOX 5 expression was observed in melanoma and non-small cell lung cancer cells; and DUOX-1 and -2 levels were low across all the cell lines examined including lung cancer cells (2,14). Interestingly, however, NOX accessory proteins p22phox and Rac1 were widely distributed at a high level in most of the human tumor cells (2). These findings strongly suggest that the NOX enzymes are distributed in a highly tissue-specific fashion in human tumor cell lines, and the studies included in the current systematic review and meta-analysis indicated that NOX 4 is predominantly expressed in the lung cancer tissue or cell line (9,22,24,30), and thus, NOX 4 may be a therapeutic target for lung cancer.
The current systematic review was designed to analyze the potential connection of NOX/DUOX and lung cancer incidence as well as effect of NOX inhibition on lung cancer cell invasion and migration. The mechanism of NOX leading to lung cancer development, however, was not designed to be reviewed in the current systematic review, which was one of the limitations of the current study. Nevertheless, there is an accumulating body of evidence indicating that NOX-dependent ROS production contributes to the oxidation of DNA bases (primarily guanine) as well as DNA strand breaks in tumor cells, by which mechanism, it causes DNA base oxidation, the most common causes of somatic mutation in human solid tumors (33). In addition, NOX/DUOX activation appears to play an important role in producing DNA damage, enhancing proliferation and modifying the invasiveness of human tumor cells, at least when examined in vitro cell culture. Moreover, interaction of NOX and antioxidant may regulate tumor microenvironment. In this regard, imbalance of NOX4 and NFE2-related factor 2 (Nrf2) may contribute to the tumor cell migration and myofibroblast differentiation, and by which mechanism, NOX2 may augment tumor cell metastasis (34,35).
The role of the NOX family in tumor biology, including studies enrolled into the current review, has primarily been examined using in vitro cell culture systems. Although studies on NOX isoforms using human tumor cell lines has provided some initial models for the study of NOX biology and expanded our understanding of the role of NOX proteins in human tumor biology, compared to the in vivo tumor tissues, significantly lower or reduced expression of individual members of the NOX family in the tumor cell lines has been noticed. In this regard, Wu et al found that a single passage of BxPC3 human pancreatic cancer cells in vivo in murine xenograft models, without exogenous cytokine exposure, up-regulated DUOX2 expression more than 20 fold, to the same level of expression in cell culture systems that having been treated with cytokines for 24 hours (36). They also found that normal pancreas had no DUOX membrane staining, while DUOX expression were demonstrable in 34 of 48 patients with chronic pancreatitis, both in the membrane and the cytoplasm of pancreatic duct cells. Furthermore, increased DUOX expression was often closely associated with areas of inflammatory cell infiltrates (36). Thus, the discordance between DUOX expression determined by IHC in human tumors and that in vitro cell culture systems of tumor cell lines may be associated with the milieu of chronic inflammation in that the culture media are often devoid of biologically active heat-labile cytokines and growth factors (37). Unfortunately, this discrepancy may have diminished their utility for understanding whether, and to what degree, the expression of NOX isoforms is associated with human tumors in the clinic. Therefore, it is crucial to study expression of NOX family using in vivo or ex vivo tumor tissues instead of in vitro culture of tumor cell lines.
In view of the apparent contribution to tumor growth by NOX isoforms both in vitro and in vivo, recent efforts have been directed towards the development of NOX inhibitors that might be applied for cancer prevention or treatment (10,22-24). Although isoform-specific NOX inhibitors have been difficult to define, it is clear that NOX inhibition by pan-pharmacologic inhibitors or RNAi strategy appears to decrease tumor growth, tumor invasion and metastasis of lung cancer cells as demonstrated by the current systematic review. While it may be reasonable to develop therapeutic agents with a broader degree of NOX inhibitor because of the expression of several NOX isoforms in human tumors, NOX 4 specific inhibitor may potentially be appropriate to develop in against lung cancer and its oxidative microenvironment in that NOX 4 is predominantly expressed in lung cells, and that pooled effect of NOX 4 suppression on lung cancer cell invasion or migration in the current systematic review demonstrated that a significant blockade of lung cancer cell invasion and migration was achieved.
There were several limitations of the current study. First, limited number of publications was available for the selected topic, and thus, both animal and clinical studies were pooled together to conduct the meta-analysis. Second, publications were heterogeneous, and publication bias may exist. Third, the mechanism of NOX leading to lung cancer development was not designed to be reviewed in the current systematic review.
Taken together, NOX/DUOX expression plays a critical role in the development of an oxidative microenvironment where in NOX provides pro-oncogenic survival signals for tumor cell proliferation and invasion through production of reactive oxygen species (ROS). The current systematic review demonstrates that up-regulation of NOX homologues that generate ROS appears to be associated with lung cancer. Inhibition of NOX isoforms, predominantly NOX 4, by pharmacologic inhibitors or RNAi strategy result in significant suppression of lung cancer cell invasion or metastasis. Thus, we anticipate that the NOXs, especially NOX4, might be a target for prevention and treatment of lung cancer. Efforts to suppress NOXs, especially NOX 4, up-regulation or to interfere with NOXs function in tumor microenvironment may become an important approach to prevent oxidative-stress-related carcinogenesis or metastasis of lung cancer.
Acknowledgements
None.
Footnote
Conflicts of Interest: The authors have no conflicts of interest to declare.
References
- Wu Y, Antony S, Meitzler JL, et al. Molecular mechanisms underlying chronic inflammation-associated cancers. Cancer Lett 2014;345:164-73. [Crossref] [PubMed]
- Roy K, Wu Y, Meitzler JL, et al. NADPH oxidases and cancer. Clin Sci (Lond) 2015;128:863-75. [Crossref] [PubMed]
- Hussain SP, Hofseth LJ, Harris CC. Radical causes of cancer. Nat Rev Cancer 2003;3:276-85. [Crossref] [PubMed]
- Bedard K, Krause KH. The NOX family of ROS-generating NADPH oxidases: physiology and pathophysiology. Physiol Rev 2007;87:245-313. [Crossref] [PubMed]
- Martyn KD, Frederick LM, von Loehneysen K, et al. Functional analysis of Nox4 reveals unique characteristics compared to other NADPH oxidases. Cell Signal 2006;18:69-82. [Crossref] [PubMed]
- Biberstine-Kinkade KJ, DeLeo FR, Epstein RI, et al. Heme-ligating histidines in flavocytochrome b(558): identification of specific histidines in gp91(phox). J Biol Chem 2001;276:31105-12. [Crossref] [PubMed]
- Meitzler JL, Ortiz de Montellano PR. Caenorhabditis elegans and human dual oxidase 1 (DUOX1) "peroxidase" domains: insights into heme binding and catalytic activity. J Biol Chem 2009;284:18634-43. [Crossref] [PubMed]
- Lambeth JD. NOX enzymes and the biology of reactive oxygen. Nat Rev Immunol 2004;4:181-9. [Crossref] [PubMed]
- Zhang C, Lan T, Hou J, et al. NOX4 promotes non-small cell lung cancer cell proliferation and metastasis through positive feedback regulation of PI3K/Akt signaling. Oncotarget 2014;5:4392-405. [Crossref] [PubMed]
- Boudreau HE, Casterline BW, Burke DJ, et al. Wild-type and mutant p53 differentially regulate NADPH oxidase 4 in TGF-β-mediated migration of human lung and breast epithelial cells. Br J Cancer 2014;110:2569-82. [Crossref] [PubMed]
- Cheng G, Cao Z, Xu X, et al. Homologs of gp91phox: cloning and tissue expression of Nox3, Nox4, and Nox5. Gene 2001;269:131-40. [Crossref] [PubMed]
- Kuroda J, Nakagawa K, Yamasaki T, et al. The superoxide-producing NAD(P)H oxidase Nox4 in the nucleus of human vascular endothelial cells. Genes Cells 2005;10:1139-51. [Crossref] [PubMed]
- Fischer H. Mechanisms and function of DUOX in epithelia of the lung. Antioxid Redox Signal 2009;11:2453-65. [Crossref] [PubMed]
- Luxen S, Belinsky SA, Knaus UG, et al. Silencing of DUOX NADPH oxidases by promoter hypermethylation in lung cancer. Cancer Res 2008;68:1037-45. [Crossref] [PubMed]
- Takeya R, Ueno N, Kami K, et al. Novel human homologues of p47phox and p67phox participate in activation of superoxide-producing NADPH oxidases. J Biol Chem 2003;278:25234-46. [Crossref] [PubMed]
- Ambasta RK, Kumar P, Griendling KK, et al. Direct interaction of the novel Nox proteins with p22phox is required for the formation of a functionally active NADPH oxidase. J Biol Chem 2004;279:45935-41. [Crossref] [PubMed]
- Lee YH, Bae YS. Phospholipase D2 downregulation induces cellular senescence through a reactive oxygen species-p53-p21Cip1/WAF1 pathway. FEBS Lett 2014;588:3251-8. [Crossref] [PubMed]
- Wu Y, Antony S, Hewitt SM, et al. Functional activity and tumor-specific expression of dual oxidase 2 in pancreatic cancer cells and human malignancies characterized with a novel monoclonal antibody. Int J Oncol 2013;42:1229-38. [PubMed]
- Griffith B, Pendyala S, Hecker L, et al. NOX enzymes and pulmonary disease. Antioxid Redox Signal 2009;11:2505-16. [Crossref] [PubMed]
- Meitzler JL, Antony S, Wu Y, et al. NADPH oxidases: a perspective on reactive oxygen species production in tumor biology. Antioxid Redox Signal 2014;20:2873-89. [Crossref] [PubMed]
- Trinchieri G. Cancer and inflammation: an old intuition with rapidly evolving new concepts. Annu Rev Immunol 2012;30:677-706. [Crossref] [PubMed]
- Vaid M, Katiyar SK. Grape seed proanthocyanidins inhibit cigarette smoke condensate-induced lung cancer cell migration through inhibition of NADPH oxidase and reduction in the binding of p22 and p47 proteins. Mol Carcinog 2015;54 Suppl 1:E61-71. [Crossref] [PubMed]
- Liu X, Pei C, Yan S, et al. NADPH oxidase 1-dependent ROS is crucial for TLR4 signaling to promote tumor metastasis of non-small cell lung cancer. Tumour Biol 2015;36:1493-502. [Crossref] [PubMed]
- Yan S, Liu G, Pei C, et al. Inhibition of NADPH oxidase protects against metastasis of human lung cancer by decreasing microRNA-21. Anticancer Drugs 2015;26:388-98. [Crossref] [PubMed]
- Moher D, Liberati A, Tetzlaff J, et al. Preferred reporting items for systematic reviews and meta-analyses: the PRISMA statement. PLoS Med 2009;6:e1000097. [Crossref] [PubMed]
- Higgins JP, Thompson SG. Quantifying heterogeneity in a meta-analysis. Stat Med 2002;21:1539-58. [Crossref] [PubMed]
- Morré DJ, Caldwell S, Mayorga A, et al. NADH oxidase activity from sera altered by capsaicin is widely distributed among cancer patients. Arch Biochem Biophys 1997;342:224-30. [Crossref] [PubMed]
- Ilonen IK, Räsänen JV, Sihvo EI, et al. Oxidative stress in non-small cell lung cancer: role of nicotinamide adenine dinucleotide phosphate oxidase and glutathione. Acta Oncol 2009;48:1054-61. [Crossref] [PubMed]
- Kelkka T, Pizzolla A, Laurila JP, et al. Mice lacking NCF1 exhibit reduced growth of implanted melanoma and carcinoma tumors. PLoS One 2013;8:e84148. [Crossref] [PubMed]
- Zhang B, Liu Z, Hu X. Inhibiting cancer metastasis via targeting NAPDH oxidase 4. Biochem Pharmacol 2013;86:253-66. [Crossref] [PubMed]
- Juhasz A, Ge Y, Markel S, et al. Expression of NADPH oxidase homologues and accessory genes in human cancer cell lines, tumours and adjacent normal tissues. Free Radic Res 2009;43:523-32. [Crossref] [PubMed]
- Doroshow JH, Juhasz A, Ge Y, et al. Antiproliferative mechanisms of action of the flavin dehydrogenase inhibitors diphenylene iodonium and di-2-thienyliodonium based on molecular profiling of the NCI-60 human tumor cell panel. Biochem Pharmacol 2012;83:1195-207. [Crossref] [PubMed]
- van Loon B, Markkanen E, Hübscher U. Oxygen as a friend and enemy: How to combat the mutational potential of 8-oxo-guanine. DNA Repair (Amst) 2010;9:604-16. [Crossref] [PubMed]
- Hecker L, Vittal R, Jones T, et al. NADPH oxidase-4 mediates myofibroblast activation and fibrogenic responses to lung injury. Nat Med 2009;15:1077-81. [Crossref] [PubMed]
- Hecker L, Logsdon NJ, Kurundkar D, et al. Reversal of persistent fibrosis in aging by targeting Nox4-Nrf2 redox imbalance. Sci Transl Med 2014;6:231ra47. [Crossref] [PubMed]
- Wu Y, Lu J, Antony S, et al. Activation of TLR4 is required for the synergistic induction of dual oxidase 2 and dual oxidase A2 by IFN-γ and lipopolysaccharide in human pancreatic cancer cell lines. J Immunol 2013;190:1859-72. [Crossref] [PubMed]
- Giard DJ. Routine heat inactivation of serum reduces its capacity to promote cell attachment. In Vitro Cell Dev Biol 1987;23:691-7. [Crossref] [PubMed]