Deficiency of Toll-like receptor 4 attenuates airway inflammation and remodeling in an ovalbumine-induced mouse asthma model
Highlight box
Key findings
• Toll-like receptor 4 (TLR4) deficiency significantly reduced inflammatory cell infiltration and the levels of Th2 cytokines.
• TLR4 deficiency markedly decreased airway mucous production, collagen deposition, and airway smooth muscle thickness.
What is known and what is new?
• Studies have indicated elevated the expression levels of TLR4 and nuclear factor kappa B (NF-κB) in the blood mononuclear cells of ovalbumin (OVA) induced asthmatic mice. Compared with OVA group, OVA + TLR4−/− group has lower levels of IL-4, IL-5 and IL-13, and less inflammation.
• New finding that TLR4 deficiency attenuates asthma airway remodeling by reducing goblet cell metaplasia, collagen deposition and airway smooth muscle thickening.
What is the implication, and what should change now?
• Targeting TLR4 may be an effective strategy for the treatment of asthma airway inflammation and airway remodeling. However, there are still many challenges in translating it into clinical practice.
Introduction
Asthma is a chronic inflammatory disease impacting the airways, affecting an estimated 334 million people worldwide and representing a significant global health concern (1). This autoimmune disease results from a complex interplay of genetic and environmental factors and is characterized by airway inflammation, persistent airway hyperresponsiveness, and airway remodeling (2). Features of airway remodeling include subepithelial fibrosis, collagen deposition, goblet cell hypertrophy, mucus hypersecretion, thickening of basement membranes, and smooth muscle hypertrophy. Inflammatory cells such as eosinophils and neutrophils also play a crucial role in the remodeling process (3-5). A shift towards Th2-dominant responses, regulated by transcription factors T-bet and GATA-binding protein 3 (GATA-3), is believed to drive the pathogenesis of asthma (6-8).
Toll-like receptors (TLRs) are pivotal components of the innate immune system, recognizing pathogen-associated molecular patterns and initiating immune responses. TLR4, in particular, is instrumental in modulating both innate and adaptive immune responses, crucial for initiating and regulating airway inflammation (9,10). It activates key inflammatory pathways such as the Jun N-terminal kinase (JNK) and nuclear factor kappa B (NF-κB) pathways, sustaining a state of chronic inflammation (11-14). Research by Roy et al. underscores the significance of the TLR4/NF-κB pathway in inflammatory diseases, suggesting that although its disruption can be beneficial, prolonged blockade might compromise host defense mechanisms (15,16). Experimental studies have demonstrated that TLR4-mediated signaling is essential for Th2 cells to respond to non-microbial protein allergens (9). In models of house dust mite (HDM)-induced experimental asthma, inhibition of TLR4 was shown to decrease airway eosinophilia and reduce bronchial hyperresponsiveness (17,18), highlighting the potential therapeutic benefits of targeting this pathway.
Extensive research has established the role of TLR4 and the MyD88-dependent pathway as principal contributors to the pathogenesis of asthma through upstream activation of NF-κB (19,20). NF-κB, a pivotal transcription factor, regulates inflammatory and immune responses by modulating Th2 cytokine production and gene expression. Studies have indicated elevated messenger RNA (mRNA) expression levels of TLR4 and NF-κB in the blood mononuclear cells of ovalbumin (OVA)-induced asthmatic mice (21-23). Moreover, the inhibition of NF-κB has been shown to attenuate allergic asthma in OVA-induced models (24-26). Recent findings by Tang et al. revealed that a TLR4 antagonist can alleviate symptoms in both upper and lower airways in a mouse model of chronic allergic rhinitis and asthma syndrome (CARAS) by downregulating CCL2 expression and reducing monocyte infiltration in nasal mucosa and lung tissues (27). Furthermore, TLR4 antagonist activity has been observed to reverse changes in lung function parameters, inflammatory cytokine levels, and circulating blood cell counts in asthmatic rats (28).
These findings have led us to hypothesize that TLR4 plays a critical role in airway inflammation and remodeling. In this study, we explore the regulatory effects of TLR4 in airway inflammation and remodeling using a TLR4 knockout (KO) mouse model of asthma. We present this article in accordance with the ARRIVE reporting checklist (available at https://jtd.amegroups.com/article/view/10.21037/jtd-24-1751/rc).
Methods
Reagents and antibodies
All primers were synthesized by Sangon Biotech. We utilized TaKaRa Ex Taq® DNA Polymerase (RR001A, TaKaRa, Kusatsu, Japan), PrimeScript™ RT reagent Kit with gDNA Eraser (RR047, Takara Bio, Inc.), and Bio-Rad Universal SYBR Green Supermix (#172-5124, Bio-Rad, Hercules, USA). Ovalbumin (A5503, Sigma-Aldrich, St. Louis, USA) and Imject™ Alum Adjuvant (Thermo Fisher Scientific, Waltham, USA) were used for sensitization. Enzyme-linked immunosorbent assay (ELISA) kits for interleukin-4, 5, 13 (IL-4, IL-5, IL-13), and interferon-gamma (IFN-γ) (Thermo Fisher Scientific) were employed. Primary antibodies for T-bet (ab307193, Abcam, Cambridge, UK), GATA-3 (ab199428, Abcam), α-smooth muscle actin (α-SMA) (C6198, Sigma-Aldrich), NF-κB (#8242, Cell Signaling Technology, Danvers, USA), inhibitor of NF-κB (IκB) (#4812, Cell Signaling Technology) and β-Actin (sc-47778, Santa Cruz, Dallas, USA) were used.
Animal and experimental design
Experiments were performed under a project license (No. GLMC202005032) granted by the Guilin Medical University Animal Ethics Committee, in compliance with institutional guidelines for the care and use of animals. Wild-type (WT) female C57BL/6J and C57BL/10ScNJGpt TLR4 KO mice (6–8 weeks) were derived from Gempharmatech. The mice were housed in a facility specifically designed to be free of pathogens, where they were subjected to a controlled 12-hour light/dark cycle, maintained at a temperature range of 20–24 ℃, and a relative humidity of 50–60%.
Mice were randomly assigned to five groups (n=6 per group): control, TLR4 KO, OVA sensitized, TLR4 KO OVA sensitized and OVA sensitized dexamethasone (DXM) intervention. Mice in the OVA groups were intraperitoneally injected with a sensitization solution of 80 µg OVA mixed with Imject™ Alum Adjuvant on days 0 and 7. Challenges with 1% OVA were conducted via nebulization for 30 minutes every other day from days 14 to 55. Controls and TLR4 KOs received 0.9% sterile saline following the same protocol, as depicted in Figure 1. On day 56, after eyeball blood collection, alveolar lavage fluid collection and lung tissue extraction, each mouse was killed by cervical dislocation. Before blood was drawn from the eye, each mouse was anesthetized with an injection of sodium pentobarbital. We selected a small sample size due to the experiment’s anticipated consistency in producing homogeneous results upon repeated trials.
Bronchoalveolar lavage fluid (BALF) and serum analysis
BALF and serum samples were collected within 24 hours of the final exposure. Mice were bled via retro-orbital puncture; plasma was centrifuged at 4 ℃, 3,500 rpm for 10 minutes, and stored at −80 ℃. BALF was retrieved by lavaging the airways with 0.9% ice-cold saline, centrifuged under similar conditions, and the cells counted. Differential cell counts were performed on Giemsa-stained smears by two independent, blinded researchers. Cytokine levels in serum were quantified using the appropriate ELISA kits.
Lung tissue staining
Following BALF collection, the left lung lobe was fixed in 4% paraformaldehyde for 24 hours, dehydrated, and embedded in paraffin. Sections (5 µm thick) were stained with hematoxylin and eosin (H&E), periodic acid-Schiff (PAS), and Masson’s trichrome (Masson). Immunostaining for GATA-3, T-bet, and α-SMA was also conducted. The extent of inflammation, mucus production, collagen deposition, and airway smooth muscle accumulation was quantified through morphometric analysis.
RNA preparation and real-time quantitative reverse transcription polymerase chain reaction (qRT-PCR)
Total RNA was extracted from the right lung lobes using Trizol, and cDNA was synthesized using the PrimeScript™ RT reagent Kit with gDNA Eraser. Quantitative PCR was conducted using the Bio-Rad Universal SYBR Green Supermix on a CFX96™ Real-Time System (BIO-RAD, Inc., MA, Singapore). Expression levels were normalized to β-actin, and relative gene expression was quantified using the comparative 2−ΔΔCq method (23).
Protein extraction and western blot assay
Proteins were extracted from lung tissues, resolved by sodium dodecyl sulfate-polyacrylamide gel electrophoresis (SDS-PAGE), and transferred to polyvinylidene fluoride (PVDF) membranes. After blocking with 1% bovine serum albumin (BSA)/5% skim milk, membranes were incubated with primary antibodies overnight at 4 ℃ and secondary antibodies for 1–2 hours at room temperature. Bands were visualized using photographic film and analyzed using ImageJ software.
Statistical analysis
No data or experiments were excluded from the analysis. Data were expressed as mean ± standard deviation (SD). Statistical significance was assessed using one-way analysis of variance followed by least significant difference (LSD) or Dunnett’s test for multiple comparisons. Data analysis was performed using SPSS 22.0. Statistical significance was indicated by P<0.05.
Results
TLR4 deficiency reduces inflammatory cell infiltration
In the context of ovalbumin (OVA)-induced asthma, our data reveal that the presence of TLR4 significantly influences the infiltration of inflammatory cells. Compared to WT mice, TLR4 KO mice exhibited a substantial reduction in both the total number of white blood cells and the count of eosinophils in the BALF, as depicted in Figure 2. Histological examinations further substantiated these findings, where H&E staining of lung sections from TLR4 KO mice showed markedly less inflammation, which was quantitatively supported by lower inflammation scores (Figure 3).
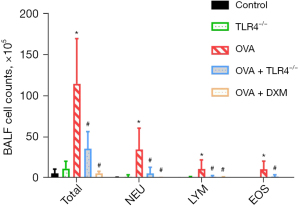
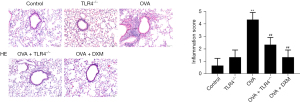
Modulation of serum cytokine levels by TLR4 deficiency
Challenging mice with OVA significantly upregulated the serum levels of Th2 cytokines (IL-4, IL-5, and IL-13), which are typically associated with allergic inflammation, while concurrently reducing the levels of the Th1 cytokine IFN-γ (Figure 4). Notably, in the OVA + TLR4−/− group and the group treated with DXM, there was a significant decrease in Th2 cytokines coupled with an enhancement of IFN-γ levels. This cytokine profile shift suggests a move towards a Th1-mediated immune response, indicating a potential mechanism through which TLR4 deficiency modulates the immune landscape in asthmatic conditions.
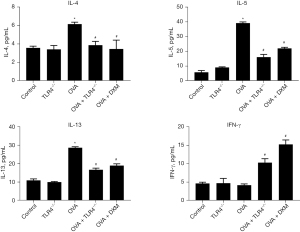
Impact of TLR4 deficiency on transcription factor mRNA and protein levels
Our qRT-PCR analysis highlighted a significant modulation of key transcription factors in response to TLR4 deficiency. In the OVA group, there was a notable decrease in the mRNA expression of the Th1 master regulator T-bet compared to controls, whereas TLR4 KO resulted in increased T-bet mRNA levels in both OVA + TLR4−/− and OVA + DXM groups (Figure 5A). Concurrently, mRNA levels of the Th2 transcription factor GATA-3 were elevated in the OVA group and significantly diminished in TLR4-deficient and DXM-treated groups. These trends were mirrored at the protein level, as demonstrated by immunohistochemistry, which showed a significant enhancement of the T-bet/GATA-3 ratio, indicative of a shift towards Th1 dominance in the immune response within the lungs of these models (Figure 5B,5C).
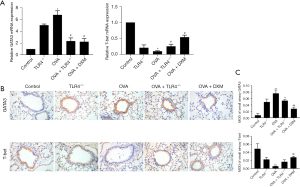
TLR4 deficiency alleviates airway remodeling by attenuating goblet cell metaplasia and collagen deposition
PAS staining used to assess goblet cell metaplasia and Masson trichrome staining employed to quantify collagen deposition both revealed that TLR4 KO significantly curtailed mucus production and collagen buildup in the lungs of OVA-treated mice. This reduction underscores the role of TLR4 in airway remodeling processes typical of asthmatic responses (Figure 6A-6D).
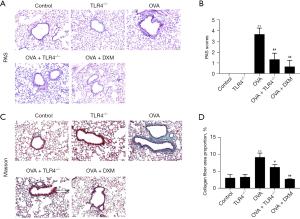
TLR4 deficiency alleviates airway remodeling by reducing airway smooth muscle thickening
Investigation into airway structural changes revealed that α-SMA expression, a definitive marker of airway smooth muscle, was significantly enhanced in the OVA group but markedly reduced in the TLR4 KO and DXM-treated groups. Image-Pro Plus 6.0 software analysis confirmed a decreased mean thickness of airway smooth muscle in these TLR4-deficient groups, highlighting the impact of TLR4 on airway remodeling dynamics (Figure 7).
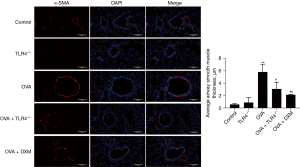
Inhibition of NF-κB pathway by TLR4 deficiency
The final aspect of our analysis focused on the signaling mechanisms impacted by TLR4 deficiency. Western blot analysis of lung tissue from the OVA-induced asthma model illustrated significant alterations in the NF-κB signaling pathway. Specifically, NF-κB protein expression was significantly decreased, while IκB protein expression was elevated in the OVA + TLR4−/− group compared to the OVA group. These findings suggest that TLR4 KO inhibits the NF-κB pathway, thereby potentially reducing the inflammatory response characteristic of asthma (Figure 8).
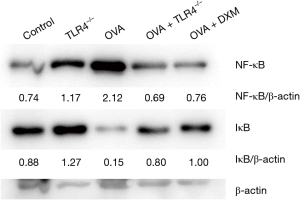
Discussion
Asthma is a widespread chronic respiratory condition fundamentally marked by airway inflammation and structural changes, often termed remodeling (29). In this study, we established an asthma mouse model by using TLR4 KO mice to observe the effects of TLR4 deletion on airway inflammation and airway remodeling in asthmatic mice, and further explored its mechanisms. Central to the inflammation are Th2 lymphocytes, which propagate the asthmatic response by releasing cytokines such as interleukin (IL)-4, IL-5, and IL-13, driving both airway hyperresponsiveness and eosinophilic inflammation (30,31). Cytokine targeting has emerged as a particularly effective strategy in asthma management, reflecting a shift toward more tailored therapeutic interventions. For instance, monoclonal antibodies targeting IL-4Rα, which mediates the activity of both IL-4 and IL-13, have been successful in reducing exacerbations and improving pulmonary function in severe asthmatics (32-34). Similarly, antagonists against IL-5Rα have shown promise in significantly reducing eosinophilic inflammation, which is a hallmark of certain asthma phenotypes, thus preventing severe asthma attacks in individuals with refractory asthma (35,36).
This study’s findings significantly extend our understanding of TLR4’s role in modulating these processes. By demonstrating that TLR4 deficiency not only reduces the infiltration of inflammatory cells but also decreases Th2 cytokine production and modifies transcription factor dynamics in the T-bet/GATA-3 axis, we highlight a potential mechanistic pathway through which TLR4 influences asthma pathogenesis (37,38). The results align with those reported by other researchers, in which TLR4 deficiency attenuates asthma inflammation. Intriguingly, activation of the TLR4 signaling pathway exacerbates asthma that is induced by Al2O3 nanoparticles (NPs) (39). In addition, inhibition of TLR4/MyD88/NF-κB signaling with targeted molecules can attenuate asthma pathology and play an important role in airways protection (40). TLR4 antagonist ameliorates combined allergic rhinitis and asthma syndrome by reducing inflammatory monocytes infiltration in an OVA-induced mouse model (27).
The treatment of chronic asthma is challenging and often involves complex therapeutic regimens, making it particularly important to control structural changes in the airways and prevent deterioration.
Targeted therapies have emerged as a particularly effective strategy for treating asthma, reflecting a shift toward more tailored therapeutic interventions. For instance, miR-15b-5p reduces airway inflammatory cells and ameliorates airway inflammation and remodeling by inhibiting the HMGB1/TLR4/IL-33 signaling pathway (41). Similarly, α2-adrenoceptor agonist Dexmedetomidine reverses the increased expression of TLR4 and its downstream signaling molecule NF-κB in lung tissue of asthmatic mice. Significantly improved airway inflammation and airway remodeling in asthmatic mice. The airway remodeling was evaluated by goblet cell hyperplasia, peribronchial collagen deposition and airway smooth muscle hyperplasia (42). High-dose Resveratrol attenuated asthma-induced airway inflammation and airway remodeling by inhibiting the HMGB1/TLR4/NF-κB signaling pathway (29). The implications of reduced mucus production, collagen deposition, and smooth muscle thickness in our TLR4 KO models suggest that targeting TLR4 could be a viable therapeutic strategy to mitigate core features of asthma airway remodeling pathology.
Our findings indicate a protective effect of TLR4 deletion in OVA-induced asthmatic mice model and elucidate the underlying mechanisms. The modulation of the NF-κB pathway by TLR4 KO add to the growing body of evidence supporting the critical role of this signaling cascade in asthma (42). The NF-κB pathway is known for its pivotal role in the regulation of immune responses, with its activation leading to the production of inflammatory cytokines and mediators that contribute to the pathophysiology of asthma (43,44). By showing that TLR4 deficiency leads to reduced NF-κB activation and increased IκB levels, this study suggests that interfering with TLR4 signaling could serve to dampen NF-κB-mediated inflammatory responses. This is consistent with observations from other inflammatory models, where TLR4 KO has been shown to mitigate inflammation and cellular stress responses, such as those induced by high-fat diets, through mechanisms involving NF-κB and JNK pathways (45). Our results presented here reinforce the potential of TLR4 as a therapeutic target for airway remodeling in asthma, suggesting that suppression of the TLR4/NF-κB signaling axis could lead to an improvement of asthma. The observed increase in IκB expression further supports the role of this pathway as a fundamental regulator of immune responses in asthma.
The comprehensive analysis of TLR4’s impact on asthma in this study underlines the protective effects of its KO, which extends beyond mere symptom management to potentially altering the disease’s underlying inflammatory mechanisms. This dual impact on both cytokine production and structural remodeling of airways provides a compelling argument for the development of TLR4-targeted therapies that could offer a more decisive approach to managing asthma.
Conclusions
In conclusion, the present study demonstrated that TLR4 deficiency attenuated asthmatic airway inflammation and airway remodeling in the OVA-induced asthmatic mice model by regulating the expression of NF-κB and IκB. Our findings provide clear evidence for targeted therapy of TLR4.
Acknowledgments
None.
Footnote
Reporting Checklist: The authors have completed the ARRIVE reporting checklist. Available at https://jtd.amegroups.com/article/view/10.21037/jtd-24-1751/rc
Data Sharing Statement: Available at https://jtd.amegroups.com/article/view/10.21037/jtd-24-1751/dss
Peer Review File: Available at https://jtd.amegroups.com/article/view/10.21037/jtd-24-1751/prf
Funding: This study was supported by
Conflicts of Interest: All authors have completed the ICMJE uniform disclosure form (available at https://jtd.amegroups.com/article/view/10.21037/jtd-24-1751/coif). The authors have no conflicts of interest to declare.
Ethical Statement: The authors are accountable for all aspects of the work in ensuring that questions related to the accuracy or integrity of any part of the work are appropriately investigated and resolved. Experiments were performed under a project license (No. GLMC202005032) granted by the Guilin Medical University Animal Ethics Committee, in compliance with institutional guidelines for the care and use of animals.
Open Access Statement: This is an Open Access article distributed in accordance with the Creative Commons Attribution-NonCommercial-NoDerivs 4.0 International License (CC BY-NC-ND 4.0), which permits the non-commercial replication and distribution of the article with the strict proviso that no changes or edits are made and the original work is properly cited (including links to both the formal publication through the relevant DOI and the license). See: https://creativecommons.org/licenses/by-nc-nd/4.0/.
References
- Papi A, Brightling C, Pedersen SE, et al. Asthma. Lancet 2018;391:783-800. [Crossref] [PubMed]
- Feng KN, Meng P, Zou XL, et al. IL-37 protects against airway remodeling by reversing bronchial epithelial-mesenchymal transition via IL-24 signaling pathway in chronic asthma. Respir Res 2022;23:244. [Crossref] [PubMed]
- Boonpiyathad T, Sözener ZC, Satitsuksanoa P, et al. Immunologic mechanisms in asthma. Semin Immunol 2019;46:101333. [Crossref] [PubMed]
- Varricchi G, Ferri S, Pepys J, et al. Biologics and airway remodeling in severe asthma. Allergy 2022;77:3538-52. [Crossref] [PubMed]
- Guida G, Riccio AM. Immune induction of airway remodeling. Semin Immunol 2019;46:101346. [Crossref] [PubMed]
- Provoost S, Maes T, Van Durme YM, et al. Decreased FOXP3 protein expression in patients with asthma. Allergy 2009;64:1539-46. [Crossref] [PubMed]
- Yagi R, Zhu J, Paul WE. An updated view on transcription factor GATA3-mediated regulation of Th1 and Th2 cell differentiation. Int Immunol 2011;23:415-20. [Crossref] [PubMed]
- Hirose K, Iwata A, Tamachi T, et al. Allergic airway inflammation: key players beyond the Th2 cell pathway. Immunol Rev 2017;278:145-61. [Crossref] [PubMed]
- Soh WT, Zhang J, Hollenberg MD, et al. Protease allergens as initiators-regulators of allergic inflammation. Allergy 2023;78:1148-68. [Crossref] [PubMed]
- Allam VSRR, Faiz A, Lam M, et al. RAGE and TLR4 differentially regulate airway hyperresponsiveness: Implications for COPD. Allergy 2021;76:1123-35. [Crossref] [PubMed]
- Laouedj M, Tardif MR, Gil L, et al. S100A9 induces differentiation of acute myeloid leukemia cells through TLR4. Blood 2017;129:1980-90. [Crossref] [PubMed]
- Li J, Tian M, Hua T, et al. Combination of autophagy and NFE2L2/NRF2 activation as a treatment approach for neuropathic pain. Autophagy 2021;17:4062-82. [Crossref] [PubMed]
- Margaritopoulos GA, Lasithiotaki I, Antoniou KM. Toll-like receptors and autophagy in interstitial lung diseases. Eur J Pharmacol 2017;808:28-34. [Crossref] [PubMed]
- Oliveira MI, Pinto ML, Gonçalves RM, et al. Adsorbed Fibrinogen stimulates TLR-4 on monocytes and induces BMP-2 expression. Acta Biomater 2017;49:296-305. [Crossref] [PubMed]
- Kuzmich NN, Sivak KV, Chubarev VN, et al. TLR4 Signaling Pathway Modulators as Potential Therapeutics in Inflammation and Sepsis. Vaccines (Basel) 2017;5:34. [Crossref] [PubMed]
- Roy A, Srivastava M, Saqib U, et al. Potential therapeutic targets for inflammation in toll-like receptor 4 (TLR4)-mediated signaling pathways. Int Immunopharmacol 2016;40:79-89. [Crossref] [PubMed]
- Dang EV, Lei S, Radkov A, et al. Secreted fungal virulence effector triggers allergic inflammation via TLR4. Nature 2022;608:161-7. [Crossref] [PubMed]
- Teufelberger AR, Nordengrün M, Braun H, et al. The IL-33/ST2 axis is crucial in type 2 airway responses induced by Staphylococcus aureus-derived serine protease-like protein D. J Allergy Clin Immunol 2018;141:549-59.e7. [Crossref] [PubMed]
- Zuo L, Lucas K, Fortuna CA, et al. Molecular Regulation of Toll-like Receptors in Asthma and COPD. Front Physiol 2015;6:312. [Crossref] [PubMed]
- Wu YF, Li ZY, Dong LL, et al. Inactivation of MTOR promotes autophagy-mediated epithelial injury in particulate matter-induced airway inflammation. Autophagy 2020;16:435-50. [Crossref] [PubMed]
- Vistain L, Van Phan H, Keisham B, et al. Quantification of extracellular proteins, protein complexes and mRNAs in single cells by proximity sequencing. Nat Methods 2022;19:1578-89. [Crossref] [PubMed]
- Hata M, Andriessen EMMA, Hata M, et al. Past history of obesity triggers persistent epigenetic changes in innate immunity and exacerbates neuroinflammation. Science 2023;379:45-62. [Crossref] [PubMed]
- Xu J, Wang Y, Chen P. Lung injury in ovalbumin-challenged asthma mice induced by high-dose PM2.5 and its mechanism. Xi Bao Yu Fen Zi Mian Yi Xue Za Zhi 2017;33:1297-302. [PubMed]
- Li HY, Meng JX, Liu Z, et al. Propofol Attenuates Airway Inflammation in a Mast Cell-Dependent Mouse Model of Allergic Asthma by Inhibiting the Toll-like Receptor 4/Reactive Oxygen Species/Nuclear Factor κB Signaling Pathway. Inflammation 2018;41:914-23. [Crossref] [PubMed]
- Zhang H, Yang N, Wang T, et al. Vitamin D reduces inflammatory response in asthmatic mice through HMGB1/TLR4/NF‑κB signaling pathway. Mol Med Rep 2018;17:2915-20. [PubMed]
- Zhang Q, Wang L, Chen B, et al. Propofol inhibits NF-κB activation to ameliorate airway inflammation in ovalbumin (OVA)-induced allergic asthma mice. Int Immunopharmacol 2017;51:158-64. [Crossref] [PubMed]
- Tang H, Li T, Han X, et al. TLR4 antagonist ameliorates combined allergic rhinitis and asthma syndrome (CARAS) by reducing inflammatory monocytes infiltration in mice model. Int Immunopharmacol 2019;73:254-60. [Crossref] [PubMed]
- Thakur VR, Beladiya JV, Chaudagar KK, et al. An anti-asthmatic activity of natural Toll-like receptor-4 antagonist in OVA-LPS-induced asthmatic rats. Clin Exp Pharmacol Physiol 2018;45:1187-97. [Crossref] [PubMed]
- Jiang H, Duan J, Xu K, et al. Resveratrol protects against asthma-induced airway inflammation and remodeling by inhibiting the HMGB1/TLR4/NF-κB pathway. Exp Ther Med 2019;18:459-66. [Crossref] [PubMed]
- Lee WWL, Puan KJ, Lee B, et al. Eosinophilic allergic rhinitis is strongly associated with the CD45RBlo subset of CD161+ Th2 cells that secretes IL-2, IL-3, IL-4, IL-5, IL-9, and IL-13. Allergy 2023;78:2794-8. [Crossref] [PubMed]
- Ferreira ACF, Szeto ACH, Clark PA, et al. Neuroprotective protein ADNP-dependent histone remodeling complex promotes T helper 2 immune cell differentiation. Immunity 2023;56:1468-1484.e7. [Crossref] [PubMed]
- Porsbjerg CM, Sverrild A, Lloyd CM, et al. Anti-alarmins in asthma: targeting the airway epithelium with next-generation biologics. Eur Respir J 2020;56:2000260. [Crossref] [PubMed]
- Khumalo J, Kirstein F, Scibiorek M, et al. Therapeutic and prophylactic deletion of IL-4Ra-signaling ameliorates established ovalbumin induced allergic asthma. Allergy 2020;75:1347-60. [Crossref] [PubMed]
- Manson ML, Säfholm J, James A, et al. IL-13 and IL-4, but not IL-5 nor IL-17A, induce hyperresponsiveness in isolated human small airways. J Allergy Clin Immunol 2020;145:808-817.e2. [Crossref] [PubMed]
- FitzGerald JM, Bleecker ER, Nair P, et al. Benralizumab, an anti-interleukin-5 receptor α monoclonal antibody, as add-on treatment for patients with severe, uncontrolled, eosinophilic asthma (CALIMA): a randomised, double-blind, placebo-controlled phase 3 trial. Lancet 2016;388:2128-41. [Crossref] [PubMed]
- Hassani M, Koenderman L. Immunological and hematological effects of IL-5(Rα)-targeted therapy: An overview. Allergy 2018;73:1979-88. [Crossref] [PubMed]
- Barnes PJ. Targeting cytokines to treat asthma and chronic obstructive pulmonary disease. Nat Rev Immunol 2018;18:454-66. [Crossref] [PubMed]
- Lambrecht BN, Hammad H, Fahy JV. The Cytokines of Asthma. Immunity 2019;50:975-91. [Crossref] [PubMed]
- Lim JO, Kim WI, Pak SW, et al. Toll-like receptor 4 is a key regulator of asthma exacerbation caused by aluminum oxide nanoparticles via regulation of NF-κB phosphorylation. J Hazard Mater 2023;448:130884. [Crossref] [PubMed]
- Ma B, Athari SS, Mehrabi Nasab E, et al. PI3K/AKT/mTOR and TLR4/MyD88/NF-κB Signaling Inhibitors Attenuate Pathological Mechanisms of Allergic Asthma. Inflammation 2021;44:1895-907. [Crossref] [PubMed]
- Liu W, Li L, Piao Y, et al. Mechanism of action of miR-15b-5p in alleviating asthma airway remodeling through the HMGB1/TLR4/IL-33 signaling axis. Int Immunopharmacol 2025;145:113753. [Crossref] [PubMed]
- Zhou Y, Du X, Wang Q, et al. Dexmedetomidine Protects against Airway Inflammation and Airway Remodeling in a Murine Model of Chronic Asthma through TLR4/NF‐κB Signaling Pathway. Mediators of Inflammation 2023;2023:3695469. [Crossref] [PubMed]
- Lertnimitphun P, Zhang W, Fu W, et al. Safranal Alleviated OVA-Induced Asthma Model and Inhibits Mast Cell Activation. Front Immunol 2021;12:585595. [Crossref] [PubMed]
- Lu X, Xu C, Yang R, et al. Ganoderic Acid A Alleviates OVA-Induced Asthma in Mice. Inflammation 2021;44:1908-15. [Crossref] [PubMed]
- Hu N, Zhang Y. TLR4 knockout attenuated high fat diet-induced cardiac dysfunction via NF-κB/JNK-dependent activation of autophagy. Biochim Biophys Acta Mol Basis Dis 2017;1863:2001-11. [Crossref] [PubMed]