The significance of periostin in the diagnosis of idiopathic pulmonary fibrosis and prediction of acute exacerbations
Highlight box
Key findings
• Periostin (POSTN) is a valuable idiopathic pulmonary fibrosis (IPF) serum biomarker with better diagnostic performance than Krebs von den Lungen-6 (KL-6), surfactant protein A (SP-A), and surfactant protein D (SP-D). A decline in the predicted diffusing capacity for carbon monoxide (DLCO%pred) and an increase in baseline KL-6 levels are risk factors for acute exacerbation in IPF patients.
What is known and what is new?
• POSTN has emerged as a research hotspot, and playing a significant role in lung tissue remodeling and fibrosis development, thereby serving as a potential IPF biomarker.
• This study adds a comparative analysis of POSTN with KL-6, SP-A, and SP-D in diagnosing IPF. It explores the correlation of these four biomarkers with lung function, high-resolution computed tomography, and pulmonary artery systolic pressure in IPF patients, as well as their ability to predict acute exacerbations of IPF (AE-IPF).
What is the implication, and what should change now?
• POSTN outperforms KL-6, SP-A, and SP-D in diagnosing IPF, having the highest sensitivity and specificity among the four serum biomarkers. POSTN, KL-6, SP-A, and SP-D are useful for diagnosing and assessing IPF. DLCO%pred and KL-6 have high predictive value for AE-IPF.
Introduction
Idiopathic pulmonary fibrosis (IPF) is a chronic, progressive interstitial lung disease characterized primarily by inflammation and fibrosis of the lung tissue. It manifests with symptoms such as dyspnoea and persistent dry cough, and in severe cases, it can lead to respiratory failure and death (1,2). Pulmonary fibrosis severely disrupts the structure of lung tissue, leading to hypoxia and ventilatory impairment. Clinically, it often manifests as a significant decline in lung function and progressive dyspnoea, with some cases accompanied by pulmonary arterial hypertension (3,4). The incidence of IPF is approximately 2–30 cases per 100,000 individuals and is increasing annually (5). The incidence of IPF increases with age and predominantly affects males. Without treatment, the median survival time is 3–5 years, with a low survival rate (6,7). In the early stages of the disease, there are no obvious symptoms. Current medications for treating IPF, such as pirfenidone and nintedanib, can only slow disease progression and have limited therapeutic efficacy (8). Early diagnosis of IPF and accurate assessment of disease progression can help IPF patients delay the progression of pulmonary fibrosis at an early stage, which is crucial for improving their quality of life and survival. Histopathological examination involves invasive procedures and carries the risk of complications (9). High-resolution computed tomography (HRCT) of the chest, when used for frequent follow-up monitoring, can increase both the financial burden and radiation exposure on patients (10). In contrast, emerging serum biomarker tests offer advantages such as rapid and simple procedures, comprehensive assessment, and cost-effectiveness, providing a new option for the clinical evaluation of IPF (11,12). Reportedly, serum biomarkers such as Krebs von den Lungen-6 (KL-6), surfactant protein-A (SP-A), and surfactant protein-D (SP-D) are involved in airway remodelling and the progression of lung tissue fibrosis, elevated in the serum of IPF patients, and associated with disease severity and survival rates (13,14). In addition to the aforementioned biomarkers, recent studies have indicated that periostin (POSTN) is a potential disease marker for IPF. POSTN promotes the proliferation of lung fibroblasts and is involved in airway development and alveolar epithelial cell repair (15). Reportedly, serum POSTN levels are associated with the degree of fibrosis, decline in lung function, and long-term prognosis in patients with IPF (16). Currently, studies on the significance of POSTN expression in IPF are limited, and direct comparative research on the aforementioned four serum biomarkers is lacking. In the present study, we evaluated the diagnostic capabilities of POSTN, SP-A, SP-D, and KL-6 for IPF by measuring their expression levels in the serum of IPF patients, pneumonia control group patients, and healthy individuals. Additionally, we examined their relationships with disease severity. The findings are compared with the aim of providing a basis for early diagnosis, disease assessment, and prediction of acute exacerbations of IPF (AE-IPF). We present this article in accordance with the STARD reporting checklist (available at https://jtd.amegroups.com/article/view/10.21037/jtd-24-1882/rc).
Methods
Study subjects
From October 2022 to October 2023, a total of 70 diagnosed patients were recruited at the Third Affiliated Hospital of Anhui Medical University (Hefei First People’s Hospital). Six patients receiving high-dose corticosteroid treatment, five patients with malignancies, one patient with autoimmune diseases, and three patients with severe hepatic or renal insufficiency were excluded. The remaining 55 patients were included in the IPF group. The specific process is illustrated in Figure 1. Additionally, 30 patients who were diagnosed with bacterial pneumonia (BP) during the same period were selected as the BP group; 30 individuals who underwent health examinations during the same period were selected as the healthy control (HC) group. The age range for patients in the IPF group was 49 to 91 years, for the BP group it was 51 to 85 years, and for the HC group it was 53 to 83 years. The distribution of sex and the mean ± standard deviation of age for the subjects in all three groups are presented in Table 1. The inclusion criteria for the IPF group were as follows: met the diagnostic criteria for IPF established by the American Thoracic Society and the European Respiratory Society; had clinical manifestations, including unexplained progressive dyspnoea and dry cough; had physical signs, including clubbing fingers and the presence of Velcro crackles in the lungs; and had reticular or honeycomb patterns in both lungs on HRCT (9). The exclusion criteria were as follows: had known factors that can cause pulmonary fibrosis, such as autoimmune diseases, granulomatous diseases, occupational/environmental exposures, or drug toxicity; had received medium to high doses of glucocorticoid therapy within the past 2 months (as this may affect immune and inflammatory responses and subsequently biomarker levels); had coexisting conditions such as malignancies, haematologic disorders, immunodeficiency diseases, or serious cardiovascular conditions; or had liver or kidney dysfunction (which may affect biomarker metabolism and clearance). We obtained the daily clinical information of patients from pulmonologists in the Department of Respiratory and Critical Care Medicine, both in the outpatient and inpatient settings. For patients with an initial diagnosis of IPF, we reviewed their HRCT and pulmonary function test results from the hospital database. After confirming that they met the inclusion criteria, we selected 55 IPF patients for blood sample collection based on a convenience sampling method. All study subjects had complete clinical data. The study was conducted in accordance with the Declaration of Helsinki (as revised in 2013). This study was approved by the Third Affiliated Hospital of Anhui Medical University (No. 2023-056-01). Written informed consent to access medical records was obtained from each patient.
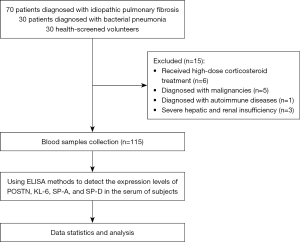
Table 1
Variables | IPF (n=55) | BP (n=30) | HC (n=30) | F/χ2 | P value |
---|---|---|---|---|---|
Age, years | 73.40±10.39 | 70.67±11.11 | 68.17±8.10 | 2.716 | 0.07 |
Sex, male/female | 34/21 | 16/14 | 15/15 | 1.271 | 0.53 |
Smoking history, yes/no | 26/29 | 12/18 | 11/19 | 1.006 | 0.61 |
BMI, kg/m2 | 21.91±2.13 | 22.08±1.75 | 22.83±1.41 | 2.384 | 0.10 |
FEV1%pred, % | 67.35±19.13 | 86.39±20.79 | 84.92±17.31 | 13.179 | <0.001 |
FVC%pred, % | 69.43±13.97 | 87.02±14.82 | 91.26±16.09 | 26.172 | <0.001 |
DLCO%pred, % | 56.73±17.87 | 85.69±13.71 | 88.79±15.69 | 50.373 | <0.001 |
PASP (mmHg) | 38.82 (24.76, 54.34) | – | – | – | – |
Data are presented as mean ± standard deviation, number, or median (first quartile, third quartile). IPF, idiopathic pulmonary fibrosis; BP, bacterial pneumonia; HC, healthy control; BMI, body mass index; FEV1%pred, the percentage of predicted forced expiratory volume in one second; FVC%pred, the percentage of predicted forced vital capacity; DLCO%pred, the percentage of the predicted diffusing capacity for carbon monoxide; PASP, pulmonary artery systolic pressure.
Measurement indicators
Measurement of serum biomarkers (POSTN, KL-6, SP-A, and SP-D)
The average interval between undergoing HRCT, pulmonary function testing, and echocardiography, and the collection of blood samples was 2.15 days (range, 0–5 days). Five mL of fasting venous blood was collected from patients in the morning. After the samples were centrifuged at 3,000 r/min in a refrigerated centrifuge, the supernatants were collected and stored in an ultralow-temperature freezer at −80 ℃. The levels of the serum biomarkers POSTN, KL-6, SP-A, and SP-D in the three groups were measured via enzyme-linked immunosorbent assay (ELISA). The POSTN and KL-6 detection kits were purchased from Wuhan Huamei Biotech Co., Ltd. (Wuhan, China), while the SP-A and SP-D kits were purchased from Beijing Solarbio Science & Technology Co., Ltd. (Beijing, China). The researchers were unable to obtain the clinical information of the patients corresponding to the serum samples. No adverse events occurred during the blood collection process. The capture antibody was added to the 96-well ELISA plate and incubated overnight at 4 ℃. After antibody immobilization, unbound antibodies were washed away with phosphate buffered saline with tween-20 (PBST) washing buffer. The wells were then blocked with phosphate buffered saline (PBS) containing 2% bovine serum albumin (BSA) at 37 ℃ for 2 hours. Following washing, standards and serum samples diluted 10, 50, and 100 times were added to each well and incubated at 37 ℃ for 2 hours, followed by another wash step. Detection antibody was then added to each well and incubated at 37 ℃ for 1 hour, followed by washing. Subsequently, enzyme conjugate was added and incubated at 37 ℃ for 30 minutes, followed by another wash step. Next, tetramethylbenzidine (TMB) substrate was added and allowed to react in the dark at 37 ℃ for 15–20 minutes. Finally, the reaction was stopped with the addition of stop solution, and the optical density (OD) values were read at 450 nm using a microplate reader. Standard curves were generated and the relative concentrations of each sample were calculated.
Pulmonary function test
The percentage of predicted forced vital capacity (FVC%pred) and the percentage of predicted forced expiratory volume in one second (FEV1%pred) were measured with a pulmonary function instrument (MasterScreen, Jaeger, Germany). Simultaneously, diffusion function testing was performed, and the percentage of the predicted diffusing capacity for carbon monoxide (DLCO%pred) was obtained via the single-breath (SB) method.
HRCT examination
HRCT examination was performed using a Revolution CT scanner (256-slice, GE Healthcare, Milwaukee, USA). The patients were placed in a supine position, and scanning was conducted from the lung apex to the diaphragm at the end of inhalation. The scanning parameters were as follows: detector coverage of 80 mm, coverage speed of 158.75 mm/s, rotation time of 0.5 s, pitch of 0.992:1, tube voltage of 100 kV, tube current 120–260 mA, scan slice thickness of 5 mm, and reconstructed slice thickness of 0.625 mm. The HRCT images obtained after scanning were reconstructed via a high spatial frequency reconstruction algorithm. The lungs were divided into three regions, upper, middle, and lower, on the basis of the levels of the carina and the lower pulmonary veins. The region above the carina level was designated the upper lung zone, the region below the lower pulmonary vein level was the lower lung zone, and the middle lung zone was located between these two levels. The total score was calculated by summing the lesion extent scores from the three regions. A higher score indicates a more severe degree of pulmonary fibrosis. For detailed methods, refer to the literature (17). The HRCT scoring was performed by two chief physicians with over ten years of experience in the radiology department. They were blinded to the clinical information of the patients corresponding to the HRCT images.
Pulmonary artery systolic pressure (PASP) measurement
Using an ultrasound diagnostic device (Philips, EPIQ 7C, Washington, USA), the tricuspid peak velocity (V) of IPF patients was measured at rest. The PASP was estimated via the tricuspid regurgitation method, with the formula PASP = 4 V² + right atrial pressure. Owing to incomplete echocardiographic data from HCs and BP patients, in this study, pulmonary artery pressure data were collected and analysed only from the IPF group.
Follow-up
Patients were followed up for 6 months to determine whether they had any acute exacerbations. This was accomplished by reviewing outpatient and inpatient medical records and conducting telephone interviews to inquire about patients’ visits to external hospitals. The clinical data of the patients were recorded. The criteria for diagnosing acute exacerbations were consistent with the AE-IPF diagnostic standards published by the AE-IPF working group, which consists of international multidisciplinary experts. These included the following: (I) past or current diagnosis of IPF; (II) severe deterioration of respiratory function within 1 month; (III) chest HRCT showing new bilateral diffuse ground-glass opacities or consolidation on top of preexisting usual interstitial pneumonia (UIP) pattern changes, such as reticular shadows or honeycombing; and (IV) exclusion of heart failure or fluid overload. The follow-up frequency was once per month (18).
Statistical analysis
Statistical analysis of the experimental data was performed via IBM SPSS 27.0 software. The Kolmogorov-Smirnov (K-S) test was used to assess the normality of the data. For normally distributed data, the mean ± standard deviation () was used, whereas for nonnormally distributed data, the median and interquartile range [M (P25, P75)] were used. Intergroup comparisons were performed via the χ2 test and analysis of variance (ANOVA). Correlation analysis within groups was conducted via Pearson or Spearman correlation analysis. Receiver operating characteristic (ROC) curves were plotted to describe the diagnostic capabilities of POSTN, KL-6, SP-A, and SP-D. P<0.05 was considered to indicate statistical significance. Univariate and multivariate binary logistic regression analyses were used to identify risk factors for AE-IPF. Patients with missing or uncertain data were excluded from subsequent data analysis.
Results
Comparison of clinical data among the three groups of subjects
The results of the comparison of clinical data among the three groups of subjects are shown in Table 1. There were no statistically significant differences in age, sex, smoking history (P=0.61), or body mass index among the three groups of subjects (P=0.10). According to the classification criteria for three pulmonary lesion regions in reference (17), there were 19 patients with single-lung region lesions, 28 patients with double-lung region lesions, and 8 patients with three-lung region lesions. Pulmonary function indicators such as FEV1%pred, FVC%pred, and DLCO%pred in the IPF group were significantly lower than those in the BP and HC groups (P<0.001). There was no significant difference in pulmonary function such as FEV1%predicted, FVC%pred, and DLCO%pred between the BP and HC groups (P=0.77, P=0.27, P=0.46). The PASP in the IPF group did not follow a normal distribution, with a median value of 38.82 mmHg (1 mmHg =0.133 kPa).
Between-group comparisons of serum POSTN, KL-6, SP-A, and SP-D concentrations
The serum levels of POSTN, KL-6, SP-A, and SP-D in the IPF group were significantly greater than those in the BP and HC groups (P<0.001). The serum concentrations of SP-A and SP-D in the BP group were greater than those in the HC group (P=0.001, P=0.04). The results are shown in Table 2 and Figure 2.
Table 2
Biomarkers | IPF | BP | HC | F value |
---|---|---|---|---|
POSTN | 98.99±26.37 | 64.15±16.10 | 58.14±13.11 | 46.567 |
KL-6 | 825.73±240.22 | 462.06±153.53 | 446.19±132.90 | 51.510 |
SP-A | 76.08±27.61 | 63.23±24.19 | 42.82±16.33 | 18.275 |
SP-D | 95.07±31.88 | 69.50±19.87 | 56.12±14.29 | 25.210 |
Data are presented as mean ± standard deviation. ANOVA of the levels of the four biomarkers in the IPF, BP, and HC groups. POSTN, periostin; KL-6, Krebs von den Lungen-6; SP-A, surfactant protein A; SP-D, surfactant protein D; IPF, idiopathic pulmonary fibrosis; BP, bacterial pneumonia; HC, healthy control; ANOVA, analysis of variance.
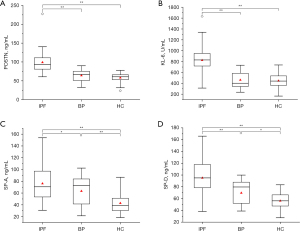
ROC curve analysis of serum POSTN, KL-6, SP-A, and SP-D for the diagnosis of IPF
The ROC curve results for distinguishing IPF patients from HCs are shown in Figure 3. The optimal cut-off values obtained from the ROC curves were as follows: POSTN: 73.84 ng/mL, KL-6: 581.69 U/mL, SP-A: 51.21 ng/mL, and SP-D: 78.34 ng/mL. All four biomarkers have good diagnostic capabilities. POSTN demonstrated the best performance in diagnosing IPF, exhibiting the highest sensitivity and specificity among the four markers. At the cut-off value of 73.84 ng/mL, POSTN showed a sensitivity of 94.5% and a specificity of 93.3%. Furthermore, POSTN had the largest area under the ROC curve (AUC =0.979), followed by KL-6 (AUC =0.925). The sensitivity, specificity, AUC, and 95% confidence intervals (95% CIs) for the four biomarkers are presented in Table 3. Table 4 shows serum POSTN, KL-6, SP-A, SP-D expression levels in relation to diagnosis of IPF.
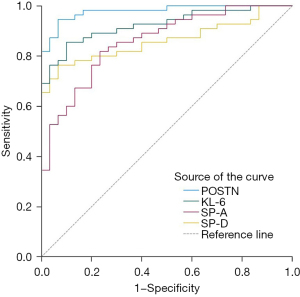
Table 3
Markers | Cut-off | Sensitivity (%) | Specificity (%) | AUC (95% CI) |
---|---|---|---|---|
POSTN | 73.84 (ng/mL) | 94.5 | 93.3 | 0.979 (0.954–1.000) |
KL-6 | 581.69 (U/mL) | 85.5 | 90.0 | 0.925 (0.871–0.979) |
SP-A | 51.21 (ng/mL) | 81.8 | 76.7 | 0.858 (0.779–0.937) |
SP-D | 78.34 (ng/mL) | 76.4 | 93.3 | 0.861 (0.784–0.939) |
AUC, area under the receiver operating characteristic curve; 95% CI, 95% confidence interval for the AUC; POSTN, periostin; KL-6, Krebs von den Lungen-6; SP-A, surfactant protein A; SP-D, surfactant protein D.
Table 4
Markers | Over cut-off | IPF | ||
---|---|---|---|---|
Positive | Negative | Total | ||
POSTN | Positive | 52 | 2 | 54 |
Negative | 3 | 28 | 31 | |
Total | 55 | 30 | ||
KL-6 | Positive | 47 | 3 | 50 |
Negative | 8 | 27 | 35 | |
Total | 55 | 30 | ||
SP-A | Positive | 45 | 7 | 52 |
Negative | 10 | 23 | 33 | |
Total | 55 | 30 | ||
SP-D | Positive | 42 | 2 | 44 |
Negative | 13 | 28 | 41 | |
Total | 55 | 30 |
IPF, idiopathic pulmonary fibrosis; POSTN, periostin; KL-6, Krebs von den Lungen-6; SP-A, surfactant protein A; SP-D, surfactant protein D.
Relationships of serum POSTN, KL-6, SP-A, and SP-D concentrations with lung function, PASP, and HRCT scores in the IPF group
In IPF patients, serum POSTN levels were negatively correlated with FEV1% predicted and DLCO% predicted. KL-6 and SP-D levels were negatively correlated with FEV1% predicted, FVC% predicted, and DLCO% predicted. The SP-A concentration was negatively correlated with the DLCO% predicted and positively correlated with PASP. The expression levels of POSTN, KL-6, and SP-A in the IPF group were significantly positively correlated with HRCT scores, with POSTN showing the highest correlation coefficient with the HRCT score. The results are shown in Figure 4 and Table 5.
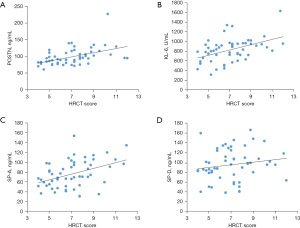
Table 5
Markers | FEV1%pred | FVC%pred | DLCO%pred | PASP | HRCT |
---|---|---|---|---|---|
POSTN | −0.345* | −0.187 | −0.396** | 0.157 | 0.472** |
KL-6 | −0.390** | −0.327* | −0.319* | 0.102 | 0.455** |
SP-A | −0.066 | −0.078 | −0.269* | 0.272* | 0.418** |
SP-D | −0.412** | −0.344* | −0.311* | 0.228 | 0.164 |
Pearson correlation analysis was used to examine the relationships of the levels of the four serum biomarkers with lung function, PASP, and HRCT scores in the IPF group. *, P<0.05 and **, P<0.01. POSTN, periostin; KL-6, Krebs von den Lungen-6; SP-A, surfactant protein A; SP-D, surfactant protein D; PASP, pulmonary artery systolic pressure; IPF, idiopathic pulmonary fibrosis; FEV1%pred, the percentage of predicted forced expiratory volume in one second; FVC%pred, the percentage of predicted forced vital capacity; DLCO%pred, the percentage of the predicted diffusing capacity for carbon monoxide; HRCT, high-resolution computed tomography.
Analysis of factors related to AE-IPF
On the basis of the follow-up results of this study, we used age, sex, smoking history, HRCT scores, lung function parameters, and four serum biomarkers as independent variables and whether patients experienced acute exacerbations within 6 months as the dependent variable (0 =no, 1 =yes). Binary logistic regression analysis was employed to identify risk factors for AE-IPF. The results of the univariate and multivariate analyses are shown in Table 6. The univariate analysis revealed that the odds ratio (OR) values for FEV1%pred and DLCO%pred were less than 1, indicating that increases in these parameters serve as protective factors against AE-IPF. Conversely, the OR values for POSTN, KL-6, and SP-A were greater than 1, suggesting that elevated levels of these serum biomarkers are risk factors for AE-IPF. The multivariate analysis revealed that the OR value for DLCO%pred was less than 1, indicating that a decrease in DLCO%pred is a risk factor for acute exacerbations. The OR value for the serum KL-6 concentration was greater than 1, indicating that increased KL-6 expression is a risk factor for AE-IPF.
Table 6
Variables | Univariate analysis | Multivariate analysis | |||
---|---|---|---|---|---|
OR (95% CI) | P | OR (95% CI) | P | ||
Age | 1.006 (0.953, 1.062) | 0.82 | – | – | |
Sex | 3.273 (0.799, 13.407) | 0.10 | – | – | |
Smoking status | 1.034 (0.315, 3.396) | 0.96 | – | – | |
HRCT score | 1.307 (0.960, 1.779) | 0.09 | – | – | |
FEV1%pred | 0.962 (0.929, 0.995) | 0.03* | – | – | |
FVC%pred | 0.967 (0.926, 1.009) | 0.12 | – | – | |
DLCO%pred | 0.905 (0.848, 0.965) | 0.002* | 0.904 (0.833, 0.980) | 0.01* | |
PASP | 1.026 (0.988, 1.065) | 0.18 | – | – | |
POSTN | 1.056 (1.019, 1.095) | 0.003* | 1.058 (0.994, 1.126) | 0.08 | |
KL-6 | 1.010 (1.004, 1.017) | 0.003* | 1.013 (1.002, 1.024) | 0.02* | |
SP-A | 1.030 (1.005, 1.055) | 0.02* | – | – | |
SP-D | 1.013 (0.993, 1.032) | 0.21 | 0.959 (0.917, 1.004) | 0.07 |
*, P<0.05. AE-IPF, acute exacerbations of idiopathic pulmonary fibrosis; OR, odds ratio; CI, confidence interval; HRCT, high-resolution computed tomography; FEV1%pred, the percentage of predicted forced expiratory volume in one second; FVC%pred, the percentage of predicted forced vital capacity; DLCO%pred, the percentage of the predicted diffusing capacity for carbon monoxide; PASP, pulmonary artery systolic pressure; POSTN, periostin; KL-6, Krebs von den Lungen-6; SP-A, surfactant protein A; SP-D, surfactant protein D.
Discussion
The histological features of IPF are associated with UIP, which is characterized by pathological proliferation of fibroblasts and myofibroblasts, as well as abnormal deposition of extracellular matrix (ECM) (19). The exact pathogenesis of IPF remains unclear. A critical early feature of the disease is damage to and apoptosis of type II alveolar epithelial cells (AEC II) (20). In addition, epithelial-mesenchymal transition (EMT) promotes an increase in myofibroblasts and ECM accumulation, contributing to fibrosis in the lungs (21). Complex interactions occur between epithelial cells, mesenchymal cells, and the ECM, along with various signalling molecules, collectively contributing to fibrotic changes in the lungs.
The progression of IPF is heterogeneous and irreversible, with lung tissue fibrosis leading to a progressive decline in lung function, hypoxemia, and even acute respiratory failure. Patients’ conditions continually worsen due to irreversible pulmonary fibrosis, resulting in a poor prognosis. Timely diagnosis and accurate assessment of the disease can facilitate early intervention and treatment, thereby improving the survival rate of IPF patients. Lung biopsy may result in complications, and the cost-effectiveness of chest HRCT is poor when it is used frequently for disease monitoring (9). Therefore, serum biomarker level testing plays an important role in the diagnosis of IPF and the assessment of disease progression.
KL-6 is a surface antigen primarily expressed on AEC II and bronchial epithelial cell membranes, and it is a high-molecular-weight mucin encoded by the MUC1 gene (22). Studies have shown that KL-6 promotes the differentiation and inhibits the apoptosis of fibroblasts in vitro, and elevated levels of KL-6 have been found in patients with IPF (23,24). In addition to IPF, KL-6 levels are also elevated in other interstitial lung diseases, whereas no increase in KL-6 expression is detected in the serum of patients with BP and chronic obstructive pulmonary disease (25,26). In the present study, the serum KL-6 levels in the IPF group were significantly greater than those in the BP and HC groups, whereas there was no significant difference in the serum KL-6 levels between the BP and HC groups (P=0.76). This may be due to abnormal senescence, apoptosis, and regeneration of AEC II during pulmonary fibrosis, which release a large amount of KL-6 into the pulmonary microcirculation. Excessive KL-6 stimulates fibroblast differentiation and ECM deposition, thereby remodelling the lung structure (27). The lack of increased KL-6 levels in patients in the BP group may be due to the absence of pathological changes in AEC II, unlike those observed in interstitial pneumonia, which does not lead to elevated serum KL-6 levels (11).
SP-A and SP-D are both components of lung surfactant and are synthesized and secreted by AEC II. Their primary function is to maintain alveolar surface tension, and they can also initiate anti-inflammatory responses in the lungs (28). SP-A and SP-D are important regulatory factors in alveolar epithelial cell apoptosis and epithelial repair. Dysregulated distribution of these proteins can lead to chronic endoplasmic reticulum stress, disrupting pulmonary homeostasis and promoting fibrosis progression (29). Elevated levels of SP-A and SP-D have been detected in the serum of patients with IPF as well as other interstitial lung diseases (11,30,31). The results of this study, showing that the serum levels of SP-A and SP-D in IPF patients are significantly greater than those in HCs and that these levels are correlated with the extent of pulmonary fibrosis (P<0.001), are consistent with those of other reports. It is possible that, after the abnormal apoptosis of AEC II caused by external factors such as acute lung injury, the expression of SP-A and SP-D increases, leading to their release into the bloodstream, where they participate in epithelial cell repair and influence the expression of transforming growth factor-β1 (TGF-β1) (32). TGF-β1 is a key profibrotic factor, and the overexpression of SP-A, SP-D, and TGF-β1, along with their complex interactions with AEC II and fibroblasts, disrupts the balance of lung tissue repair and impacts the progression of pulmonary fibrosis (32). In addition, Hamai et al. reported that the serum levels of SP-A and SP-D also increased in patients with pneumonia (33). This study similarly revealed an increase in SP-A and SP-D expression in the BP group. After the onset of an inflammatory response in the lungs, the expression of SP-A and SP-D increases, and these proteins migrate from alveolar or bronchial cells into the bloodstream, where they regulate the phagocytosis of pathogens by macrophages and the clearance of damaged or apoptotic lung cells (34,35).
POSTN is an ECM protein that was initially identified in osteoblasts and named POSTN because of its preferential expression in connective tissues such as the periosteum and periodontal ligament (36,37). POSTN can interact with αVβ3, αVβ5, and α6β4 integrins on the cell membrane and is extensively involved in tissue remodelling, fibrosis, inflammation, and tumour development. It is a potential biomarker (38). In IPF, POSTN participates in lung fibrosis by stimulating fibroblast proliferation and differentiation, inducing the EMT process, and promoting collagen fibre cross-linking (38,39). It has been reported that increased POSTN expression can occur in bleomycin-induced lung fibrosis models and that there is specific POSTN expression in the lung tissue of IPF patients (11). Ono et al. reported that serum POSTN levels are related to the degree of fibrosis, lung function decline, and long-term prognosis in IPF patients, suggesting its potential as a biomarker for relevant assessments (16). The results of this study revealed that serum POSTN levels in the IPF group were significantly greater than those in the BP and HC groups (P<0.001), were positively correlated with HRCT scores, and were negatively correlated with FEV1%pred and DLCO%pred, indicating that POSTN is closely related to the degree of lung fibrosis. These findings suggest that POSTN may promote the expression of fibrogenic factors such as connective tissue growth factor or induce EMT by activating the TGF-β/Smad signalling pathway, thereby activating fibroblasts and promoting their transformation into myofibroblasts (15). In addition, overexpressed POSTN may also catalyse the molecular-level cross-linking of collagen fibres through lysyl oxidase (LOX) activation, leading to ECM deposition and promoting the progression of lung fibrosis (40).
In addition, relatively few studies have investigated POSTN as a serum biomarker for IPF, and most of the previous studies were conducted independently without comparing POSTN levels with KL-6, SP-A, and SP-D levels. In the present study, the value of these four biomarkers for diagnosing and assessing the severity of IPF were directly compared. POSTN and KL-6 can accurately differentiate between IPF, BP, and HC groups, indicating that both have high predictive value. ELISA results show that POSTN performs better than KL-6, SP-A, and SP-D in diagnosing IPF. Specifically, POSTN has a higher AUC (0.979), sensitivity (94.5%), and specificity (93.3%) compared to KL-6 (AUC: 0.925, sensitivity: 85.5%, specificity: 90.0%). In comparison, SP-A and SP-D have slightly lower abilities to differentiate IPF, with sensitivities of 81.8% and 76.4%, respectively, which are lower than those of POSTN and KL-6. This suggests that POSTN may serve as a more sensitive and accurate biomarker for IPF. This advantage is beneficial in clinical practice for the early detection of IPF patients, enabling timely intervention and treatment. POSTN, KL-6, and SP-A levels were significantly positively correlated with HRCT scores in IPF patients, suggesting that these three markers are highly useful for assessing the degree of lung fibrosis. Additionally, KL-6 and SP-D levels were significantly negatively correlated with FEV1%pred, FVC%pred, and DLCO%pred, whereas POSTN was significantly negatively correlated with FEV1%pred and DLCO%pred, indicating that all three markers have some value in assessing lung function status in IPF patients. Only SP-A levels were positively correlated with PASP, suggesting that SP-A has some predictive value for pulmonary hypertension and assessing heart function in IPF patients.
As the disease progresses, IPF patients may experience acute respiratory function deterioration of unknown cause, defined as AE-IPF, which is similar to acute respiratory distress syndrome (ARDS) (18,41). AE-IPF progresses rapidly and is often accompanied by type I respiratory failure, with a very poor prognosis (42). This study analysed the risk factors for AE-IPF in IPF patients. Logistic regression analysis revealed that a decrease in DLCO%pred and an increase in baseline serum KL-6 levels are risk factors for AE-IPF. A decreased DLCO%pred reflects significant impairment in gas exchange due to severe fibrosis and alveolar-capillary membrane disruption. This condition reduces pulmonary reserve, making IPF patients more susceptible to acute exacerbations (43). When IPF patients have high serum KL-6 levels and decreased DLCO%pred, severe lung fibrosis often occurs, increasing their susceptibility to acute respiratory failure and other AE-IPF symptoms. Clinically, close monitoring of both markers, along with timely supportive and pharmacological treatment for IPF patients, can improve prognosis.
Conclusions
In summary, POSTN is a serum biomarker with high discriminatory power for IPF, showing better diagnostic performance than KL-6, SP-A, and SP-D. Both POSTN and KL-6 can accurately distinguish IPF patients from BP and HC patients. Our study also revealed that POSTN, KL-6, SP-A, and SP-D are related to disease progression and can be used to assess the extent of fibrosis in IPF patients. Additionally, our study revealed that a decrease in DLCO%pred and an increase in baseline serum KL-6 levels are risk factors for AE-IPF, emphasizing the need to closely monitor these two indicators to manage potential AE-IPF. However, owing to the small number of patients included in this study, the diagnostic capabilities and specificity of the serum biomarkers cannot be fully evaluated. Future studies with larger, multicenter cohorts are needed to further clarify the discriminatory power of POSTN, KL-6, SP-A, and SP-D as biomarkers for IPF.
Acknowledgments
English of this manuscript was edited by AJE (https://www.aje.cn/).
Footnote
Reporting Checklist: The authors have completed the STARD reporting checklist. Available at https://jtd.amegroups.com/article/view/10.21037/jtd-24-1882/rc
Data Sharing Statement: Available at https://jtd.amegroups.com/article/view/10.21037/jtd-24-1882/dss
Peer Review File: Available at https://jtd.amegroups.com/article/view/10.21037/jtd-24-1882/prf
Funding: This work was supported by
Conflicts of Interest: All authors have completed the ICMJE uniform disclosure form (available at https://jtd.amegroups.com/article/view/10.21037/jtd-24-1882/coif). The authors have no conflicts of interest to declare.
Ethical Statement: The authors are accountable for all aspects of the work in ensuring that questions related to the accuracy or integrity of any part of the work are appropriately investigated and resolved. The study was conducted in accordance with the Declaration of Helsinki (as revised in 2013). This study was approved by the Third Affiliated Hospital of Anhui Medical University (No. 2023-056-01). Written informed consent to access medical records was obtained from each patient.
Open Access Statement: This is an Open Access article distributed in accordance with the Creative Commons Attribution-NonCommercial-NoDerivs 4.0 International License (CC BY-NC-ND 4.0), which permits the non-commercial replication and distribution of the article with the strict proviso that no changes or edits are made and the original work is properly cited (including links to both the formal publication through the relevant DOI and the license). See: https://creativecommons.org/licenses/by-nc-nd/4.0/.
References
- Richeldi L, Azuma A, Cottin V, et al. Trial of a Preferential Phosphodiesterase 4B Inhibitor for Idiopathic Pulmonary Fibrosis. N Engl J Med 2022;386:2178-87. [Crossref] [PubMed]
- Chen T, Zeng C. Compare three diagnostic criteria of progressive pulmonary fibrosis. J Thorac Dis 2024;16:1034-43. [Crossref] [PubMed]
- Kishaba T, Nagano H, Nei Y, et al. Clinical characteristics of idiopathic pulmonary fibrosis patients according to their smoking status. J Thorac Dis 2016;8:1112-20. [Crossref] [PubMed]
- Esposito DB, Lanes S, Donneyong M, et al. Idiopathic Pulmonary Fibrosis in United States Automated Claims. Incidence, Prevalence, and Algorithm Validation. Am J Respir Crit Care Med 2015;192:1200-7. [Crossref] [PubMed]
- Harari S, Madotto F, Caminati A, et al. Epidemiology of Idiopathic Pulmonary Fibrosis in Northern Italy. PLoS One 2016;11:e0147072. [Crossref] [PubMed]
- Alhamad EH. Clinical characteristics and survival in idiopathic pulmonary fibrosis and connective tissue disease-associated usual interstitial pneumonia. J Thorac Dis 2015;7:386-93. [PubMed]
- Selvarajah B, Platé M, Chambers RC. Pulmonary fibrosis: Emerging diagnostic and therapeutic strategies. Mol Aspects Med 2023;94:101227. [Crossref] [PubMed]
- Saito S, Alkhatib A, Kolls JK, et al. Pharmacotherapy and adjunctive treatment for idiopathic pulmonary fibrosis (IPF). J Thorac Dis 2019;11:S1740-54. [Crossref] [PubMed]
- Raghu G, Remy-Jardin M, Richeldi L, et al. Idiopathic Pulmonary Fibrosis (an Update) and Progressive Pulmonary Fibrosis in Adults: An Official ATS/ERS/JRS/ALAT Clinical Practice Guideline. Am J Respir Crit Care Med 2022;205:e18-47. [Crossref] [PubMed]
- Cox IA, de Graaff B, Ahmed H, et al. The economic burden of idiopathic pulmonary fibrosis in Australia: a cost of illness study. Eur J Health Econ 2023;24:1121-39. [Crossref] [PubMed]
- Drakopanagiotakis F, Wujak L, Wygrecka M, et al. Biomarkers in idiopathic pulmonary fibrosis. Matrix Biol 2018;68-69:404-21. [Crossref] [PubMed]
- Stainer A, Faverio P, Busnelli S, et al. Molecular Biomarkers in Idiopathic Pulmonary Fibrosis: State of the Art and Future Directions. Int J Mol Sci 2021;22:6255. [Crossref] [PubMed]
- Wang C, Wang Q, Liu T, et al. Krebs von den Lungen-6 (KL-6) as a diagnostic marker for pulmonary fibrosis: A systematic review and meta-analysis. Clin Biochem 2023;114:30-8. [Crossref] [PubMed]
- Xue M, Guo Z, Cai C, et al. Evaluation of the Diagnostic Efficacies of Serological Markers KL-6, SP-A, SP-D, CCL2, and CXCL13 in Idiopathic Interstitial Pneumonia. Respiration 2019;98:534-45. [Crossref] [PubMed]
- O'Dwyer DN, Moore BB. The role of periostin in lung fibrosis and airway remodeling. Cell Mol Life Sci 2017;74:4305-14. [Crossref] [PubMed]
- Ono J, Takai M, Kamei A, et al. Pathological Roles and Clinical Usefulness of Periostin in Type 2 Inflammation and Pulmonary Fibrosis. Biomolecules 2021;11:1084. [Crossref] [PubMed]
- Yamamoto Y, Miki K, Tsujino K, et al. Oscillometry and computed tomography findings in patients with idiopathic pulmonary fibrosis. ERJ Open Res 2020;6:00391-2020. [Crossref] [PubMed]
- Collard HR, Ryerson CJ, Corte TJ, et al. Acute Exacerbation of Idiopathic Pulmonary Fibrosis. An International Working Group Report. Am J Respir Crit Care Med 2016;194:265-75. [Crossref] [PubMed]
- Richeldi L, Collard HR, Jones MG. Idiopathic pulmonary fibrosis. Lancet 2017;389:1941-52. [Crossref] [PubMed]
- Singh S, Natalini JG, Segal LN. Lung microbial-host interface through the lens of multi-omics. Mucosal Immunol 2022;15:837-45. [Crossref] [PubMed]
- Salton F, Volpe MC, Confalonieri M. Epithelial-Mesenchymal Transition in the Pathogenesis of Idiopathic Pulmonary Fibrosis. Medicina (Kaunas) 2019;55:83. [Crossref] [PubMed]
- Gao Y, Du T, Yang L, et al. Research progress of KL-6 in respiratory system diseases. Crit Rev Clin Lab Sci 2024;61:599-615. [Crossref] [PubMed]
- d'Alessandro M, Bergantini L, Cameli P, et al. Krebs von den Lungen-6 as a biomarker for disease severity assessment in interstitial lung disease: a comprehensive review. Biomark Med 2020;14:665-74. [Crossref] [PubMed]
- Cho EJ, Hong J, Hyun J, et al. Usefulness and performance evaluation of serum KL-6 and SP-A assays in healthy individuals and patients with interstitial lung disease. Clin Biochem 2023;118:110609. [Crossref] [PubMed]
- Okamoto T, Fujii M, Furusawa H, et al. The usefulness of KL-6 and SP-D for the diagnosis and management of chronic hypersensitivity pneumonitis. Respir Med 2015;109:1576-81. [Crossref] [PubMed]
- Xu L, Bian W, Gu XH, et al. Differing Expression of Cytokines and Tumor Markers in Combined Pulmonary Fibrosis and Emphysema Compared to Emphysema and Pulmonary Fibrosis. COPD 2017;14:245-50. [Crossref] [PubMed]
- Sánchez-Díez S, Munoz X, Ojanguren I, et al. YKL-40 and KL-6 Levels in Serum and Sputum of Patients Diagnosed With Hypersensitivity Pneumonitis. J Allergy Clin Immunol Pract 2022;10:2414-23. [Crossref] [PubMed]
- Mulugeta S, Nureki S, Beers MF. Lost after translation: insights from pulmonary surfactant for understanding the role of alveolar epithelial dysfunction and cellular quality control in fibrotic lung disease. Am J Physiol Lung Cell Mol Physiol 2015;309:L507-25. [Crossref] [PubMed]
- Katzen J, Beers MF. Contributions of alveolar epithelial cell quality control to pulmonary fibrosis. J Clin Invest 2020;130:5088-99. [Crossref] [PubMed]
- Doubková M, Karpíšek M, Mazoch J, et al. Prognostic significance of surfactant protein A, surfactant protein D, Clara cell protein 16, S100 protein, trefoil factor 3, and prostatic secretory protein 94 in idiopathic pulmonary fibrosis, sarcoidosis, and chronic pulmonary obstructive disease. Sarcoidosis Vasc Diffuse Lung Dis 2016;33:224-34. [PubMed]
- Györfi AH, Filla T, Dickel N, et al. Performance of serum biomarkers reflective of different pathogenic processes in systemic sclerosis-associated interstitial lung disease. Rheumatology (Oxford) 2024;63:962-9. [Crossref] [PubMed]
- Confalonieri P, Volpe MC, Jacob J, et al. Regeneration or Repair? The Role of Alveolar Epithelial Cells in the Pathogenesis of Idiopathic Pulmonary Fibrosis (IPF). Cells 2022;11:2095. [Crossref] [PubMed]
- Hamai K, Iwamoto H, Ishikawa N, et al. Comparative Study of Circulating MMP-7, CCL18, KL-6, SP-A, and SP-D as Disease Markers of Idiopathic Pulmonary Fibrosis. Dis Markers 2016;2016:4759040. [Crossref] [PubMed]
- Guagliardo R, Pérez-Gil J, De Smedt S, et al. Pulmonary surfactant and drug delivery: Focusing on the role of surfactant proteins. J Control Release 2018;291:116-26. [Crossref] [PubMed]
- Dare A, King SD, Chen SY. Surfactant protein A promotes western diet-induced hepatic steatosis and fibrosis in mice. Sci Rep 2024;14:7464. [Crossref] [PubMed]
- Okamoto M, Izuhara K, Ohta S, et al. Ability of Periostin as a New Biomarker of Idiopathic Pulmonary Fibrosis. Adv Exp Med Biol 2019;1132:79-87. [Crossref] [PubMed]
- Uchida M, Shiraishi H, Ohta S, et al. Periostin, a matricellular protein, plays a role in the induction of chemokines in pulmonary fibrosis. Am J Respir Cell Mol Biol 2012;46:677-86. [Crossref] [PubMed]
- Yoshihara T, Nanri Y, Nunomura S, et al. Periostin plays a critical role in the cell cycle in lung fibroblasts. Respir Res 2020;21:38. [Crossref] [PubMed]
- Alzobaidi N, Rehman S, Naqvi M, et al. Periostin: A Potential Biomarker and Therapeutic Target in Pulmonary Diseases. J Pharm Pharm Sci 2022;25:137-48. [Crossref] [PubMed]
- Carpagnano GE, Soccio P, Scioscia G, et al. The Potential Role of Airways Periostin in the Clinical Practice of Patients Affected by Idiopathic Pulmonary Fibrosis. Rejuvenation Res 2021;24:302-6. [Crossref] [PubMed]
- Luo X, Xiang F. Acute exacerbation of idiopathic pulmonary fibrosis a narrative review primary focus on treatments. J Thorac Dis 2024;16:4727-41. [Crossref] [PubMed]
- Biondini D, Balestro E, Sverzellati N, et al. Acute exacerbations of idiopathic pulmonary fibrosis (AE-IPF): an overview of current and future therapeutic strategies. Expert Rev Respir Med 2020;14:405-14. [Crossref] [PubMed]
- Lee H, Kim SY, Park YS, et al. Prognostic implication of 1-year decline in diffusing capacity in newly diagnosed idiopathic pulmonary fibrosis. Sci Rep 2024;14:8857. [Crossref] [PubMed]