Physical activity after video-assisted thoracoscopic lung resection following an enhanced recovery after surgery-based protocol: first data from a prospective observational study
Highlight box
Key findings
• Chest tube removal has a major impact on mobilization after video-assisted thoracoscopic (VATS) lung resection.
What is known and what is new?
• Data on postoperative mobility after VATS lung resections are largely missing.
• This study adds baseline data on postoperative mobility in an enhanced recovery after surgery setting.
What is the implication, and what should change now?
• Further studies are needed to determine what level of physical activity is required to improve patient outcomes.
Introduction
Background
Early patient mobilization is a key component of guidelines for enhanced recovery after surgery (ERAS) (1). The defined goal is to actively prevent immobilization, which is associated with general physical deconditioning and an increased risk of potentially severe postoperative complications, in particular pneumonia and venous thromboembolic events (2-4). Surprisingly, data on whether early mobilization prevents these adverse events in thoracic surgery remain scarce. Existing studies are of limited quality, and their results are contradictory (5-7).
Rationale and knowledge gap
Recommendations for early mobilization after lung resection are based on pathophysiological considerations and on data derived from other surgical disciplines, e.g., colorectal surgery (6,8).
There are currently no recommendations as to the extent and type of mobilization that would benefit thoracic surgery patients. This is due to insufficient baseline data on the postoperative physical activity (PPA) of patients after thoracic procedures.
Objective
The Pulmonary Function after Thoracic Surgery (PATHOS) trial was designed as a prospective, single-arm, single-center observational study to obtain baseline data for PPA and spirometry during the early postoperative course after video-assisted thoracoscopic (VATS) lung resection in an ERAS-based setting. Here, we present first data from this study regarding PPA. We present this article in accordance with the STROBE reporting checklist (available at https://jtd.amegroups.com/article/view/10.21037/jtd-2024-2166/rc) (9).
Methods
Study design and registration
PATHOS was a prospective, single-arm, single-center observational study registered (#DRKS00028734) with the primary German World Health Organization (WHO) Clinical Trials Register, on April 20, 2022.
Patients
The study was conducted in accordance with the Declaration of Helsinki and its subsequent amendments. Institutional review board approval (#2022-16379) was obtained from the ethics commission of the State Chamber of Physicians in Rhineland-Palatinate in Mainz, Germany, on March 21, 2022. Written informed consent was obtained from all patients prior to inclusion.
Patients were recruited between April 2022 and July 2023 at the Department of Thoracic Surgery, Center for Thoracic Diseases, University Medical Center, Mainz, Germany. Patients were approached for consent during their preoperative visit in one outpatient clinic. To be eligible, patients must have been able to understand the study, ≥18 years of age, and scheduled for elective thoracoscopic lung resection. The surgical procedures included atypical (single or multiple wedge) resections and anatomical resections (segmentectomies, lobectomies). Patients with a severely impaired mental state or language problems, participation in concurrent interventional trials, or a predictable lack of compliance (e.g., an inability to walk) were excluded. Patients with emergency surgery were also excluded.
ERAS-based treatment protocol
All participants were treated along a standardized clinical pathway based on the recommendations of the ERAS Society and the European Society of Thoracic Surgeons (ESTS) for ERAS (1). Our institutional ERAS protocol has been published before (10). In brief, it comprises 22 key ERAS features covering the preoperative, intraoperative, and postoperative phase and includes preoperative counseling, no routine administration of sedatives prior to anesthesia, prevention of hypothermia, lung protective ventilation, and a fluid management aiming for euvolemia while avoiding intravenous fluids. As a standard, we use a uniportal VATS approach when feasible and perform postoperative analgesia using a paravertebral block catheter in combination with oral acetaminophen and nonsteroidal anti-inflammatory drugs. After placement of the paravertebral block catheter, a bolus of 15–20 mL of ropivacaine solution (0.375%) is given and a continuous infusion is maintained at rates of 8–10 mL per hour throughout the procedure and postoperative period. Chest tube management comprises the use of a single, small bore chest tube connected to a digital chest drainage system without routine use of suction. All patients receive daily incentives for mobilization and are encouraged to spend more time out of bed than in bed during the day. All patients were supported in mobilization by nursing staff on a daily basis. Additionally, all patients received specialized physiotherapy and/or respiratory therapy throughout their hospital stay. This included general mobilization (assisted walking), guided use of respiratory training devices (e.g., Y-breathing trainer), gait training, and stair climbing training.
Data collection
Immediately after surgery, the research team fitted an activity tracker (Beurer AS 80, Beurer GmbH, Ulm, Germany) to the patients’ wrist. The daily number of steps and the daily minutes of physical activity were recorded until discharge or for a maximum of 5 postoperative days (PODs). Only days with complete tracking data (i.e., 24 hours) were included in our data analysis. Clinical data were acquired during daily study visits from the day of surgery until discharge or until POD 5. All study visits were performed by the same certified physiotherapist (M.F.). The thoracic morbidity and mortality score (TM&M) was used to grade surgical complications (11). Major morbidity was defined as complications ≥ grade 3; mortality was recorded as in-hospital mortality.
Statistical analysis
For quantitative variables, the median and interquartile range are presented. Qualitative variables are shown as absolute numbers and relative frequencies. To analyze binary variables in two dependent samples, McNemar’s test was used. Analysis of continuous variables in two dependent samples was carried out using the Wilcoxon signed rank test or the paired Student’s t-test, as appropriate. Analysis of continuous variables in three or more dependent samples was carried out using Friedman’s two-way analysis of variance (ANOVA) by ranks or repeated measures ANOVA with a Greenhouse-Geisser correction, as appropriate. For post-hoc pairwise comparisons, significance values were adjusted by the Bonferroni correction for multiple tests. A test result was considered statistically significant if P<0.05. All statistical tests were two-tailed. A complete-case approach was used for missing data. Analyses were performed using SPSS Statistics software (version 29.0, IBM, Armonk, NY, USA).
Results
Study population
Figure 1 shows a flowchart of the study population. A total of 129 patients were screened between April 21, 2022, and July 5, 2023. Out of these, 71 patients agreed to participate, and 68 patients were assessed with full tracking data from POD 1 until discharge or until POD 5. After excluding patients with conversion from VATS to thoracotomy (n=6), tracking data of 62 patients with thoracoscopic lung resection were evaluated in this present analysis.
Preoperative baseline characteristics
Table 1 provides the patients’ baseline characteristics. The median patient age was 64 years. Most (61.3%) patients were categorized as physical status class 3 according to the American Society of Anesthesiologists (ASA). A large majority (75.8%) of patients were current or former smokers, with a median of 30 pack years. The median predicted values for forced expiratory volume in 1 second (FEV1) and for diffusing capacity of the lungs for carbon monoxide (DLCO) were 87.5% and 72.5%, respectively. Besides abnormal lung function tests, arterial hypertension (48.4%) and coronary artery disease (14.5%) emerged as the most common comorbidities.
Table 1
Variables | Data (n=62) | Missing values |
---|---|---|
Sex | 0 | |
Female | 33 (53.2) | |
Male | 29 (46.8) | |
Age (years) | 64 [55–71] | 0 |
Body mass index (kg/m2) | 24.9 [21.6–29.0] | 0 |
Pulmonary function tests | ||
FEV1 (%) | 87.5 [74.8–101.0] | 0 |
DLCO (%) | 72.5 [59.5–84.0] | 4 |
Smoking | 0 | |
Never | 15 (24.2) | |
Currently | 15 (24.2) | |
Prior | 32 (51.6) | |
Pack years | 30 [18–40] | 1 |
ASA physical status classification | 0 | |
2 | 24 (38.7) | |
3 | 38 (61.3) | |
Medical history | ||
Hypertension | 30 (48.4) | 0 |
Coronary artery disease | 9 (14.5) | 0 |
Chronic renal disease | 5 (8.1) | 0 |
Chronic liver disease | 2 (3.2) | 0 |
Diabetes | 4 (6.5) | 0 |
Preoperative chemotherapy | 2 (3.2) | 0 |
Regular use of analgesics | 7 (11.3) | 0 |
Previous lung resection | 12 (19.4) | 0 |
Symptoms | ||
Dyspnea | 13 (21.0) | 0 |
Coughing | 14 (22.6) | 0 |
Weight loss | 9 (14.5) | 0 |
Laboratory data | ||
Sodium (mmol/L) | 141 [140–143] | 1 |
Potassium (mmol/L) | 4.2 [3.9–4.5] | 1 |
Creatinine (mg/dL) | 0.85 [0.71–0.97] | 0 |
Hb (g/dL) | 14.0 [13.4–14.9] | 0 |
Thrombocytes (/nL) | 228 [181–272] | 0 |
Leucocytes (×109/L) | 6.8 [5.6–8.4] | 0 |
CRP (mg/L) | 2.3 [0.7–5.4] | 0 |
Albumin (g/L) | 42 [39–43] | 7 |
INR | 1.0 [1.0–1.0] | 2 |
Bilirubin (mg/dL) | 0.5 [0.4–0.8] | 3 |
ASAT (U/L) | 27 [21–33] | 3 |
ALAT (U/L) | 22 [16–32] | 3 |
GGT (U/L) | 30 [22–53] | 4 |
ALP (U/L) | 84 [66–99] | 4 |
Data are presented as n (%) or median [IQR]. ALAT, alanine aminotransferase; ALP, alkaline phosphatase; ASA, American Society of Anesthesiologists; ASAT, aspartate aminotransferase; CRP, C-reactive protein; DLCO, diffusing capacity of the lungs for carbon monoxide; FEV1, forced expiratory volume in 1 second; GGT, gamma-glutamyltransferase; Hb, hemoglobin; INR, international normalized ratio; IQR, interquartile range.
Surgical procedures
Data on surgery are presented in Table 2. Of all patients who underwent VATS lung resection, 51.6% had anatomical resections (32.3% lobectomy, 19.4% segmental resection), and 48.4% had atypical (wedge) resections. At the end of the surgery, all study participants received one chest tube connected to a digital chest drainage system at a pressure of −8 cmH2O. The most used chest tube size in this study was 18 French (74.2%). Here, 95.2% of patients had a paravertebral block catheter placed intraoperatively under direct visual control of the surgeon. The median surgery time was 102 minutes. The median chest tube duration and the median length of hospital stay were 2 and 4 days, respectively. The pathological report confirmed malignant underlying disease in 75.8% of cases.
Table 2
Variables | Data (n=62) | Missing values |
---|---|---|
Lung resection | 0 | |
Lobectomy | 20 (32.3) | |
Segmentectomy | 12 (19.4) | |
Wedge resection | 30 (48.4) | |
Number of chest tubes | 0 | |
1 | 62 (100.0) | |
Chest tube pressure | 0 | |
−8 cmH2O | 62 (100.0) | |
Chest drainage system | 0 | |
Digital | 62 (100.0) | |
Chest tube size (French) | 0 | |
14 | 4 (6.5) | |
16 | 4 (6.5) | |
18 | 46 (74.2) | |
20 | 1 (1.6) | |
24 | 7 (11.3) | |
Regional anesthesia | 0 | |
Paravertebral block | 59 (95.2) | |
None | 3 (4.8) | |
Surgical outcomes | 0 | |
Length of surgery (minutes) | 102 [64–185] | |
Chest tube duration (days) | 2 [2–3] | |
Length of stay (days) | 4 [3–6] | |
Morbidity and mortality | 0 | |
TM&M grade >2 | 0 | |
Adverse events | 0 | |
Serious adverse events | 0 | |
Histology | 0 | |
Benign | 15 (24.2) | |
Malign | 47 (75.8) | |
Malignancy type | n=47 | 0 |
Primary | 27 (57.4) | |
Metastasis | 20 (42.6) |
Data are presented as n (%) or median [IQR]. IQR, interquartile range; TM&M, thoracic morbidity and mortality score.
Morbidity and adverse events
Two patients were unable to participate in the trial after initial study inclusion and had to be subsequently excluded from the analysis on POD 1. One of these patients suffered a grade 4b complication (postoperative hemorrhage), another patient was quarantined according to the German Infection Protection Act, which impaired this individual’s mobility options. No cases of major morbidity or mortality were recorded within the cohort undergoing VATS tracking data analysis. No adverse or serious adverse events related to the study interventions were observed.
PPA
The number of steps and minutes of PPA per day are shown in Table 3. The median number of steps on POD 1 was 247 [34–616] and increased to 4,455 [2,099–5,514] on POD 5. The median duration of physical activity on POD 1 was 2 [0–4] minutes and increased to 37 [15–48] minutes on POD 5. Figure 2 offers boxplots for the daily number of steps and the duration of PPA. For a total of 11 (17.7%) patients, zero steps were recorded on POD 1.
Table 3
Variables | POD 1 | POD 2 | POD 3 | POD 4 | POD 5 | P value |
---|---|---|---|---|---|---|
Patients | 62 | 60 | 38 | 24 | 11 | |
Steps | 247 [34–616] | 981 [460–1,994] | 1,816 [504–2,472] | 2,238 [943–3,048] | 4,455 [2,099–5,514] | <0.001 |
Minutes | 2 [0–4] | 7.5 [3–16] | 13 [3–20] | 18 [7–28] | 37 [15–48] | <0.001 |
Data are presented as number or median [IQR]. IQR, interquartile range; POD, postoperative day; PPA, postoperative physical activity.
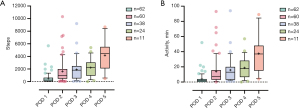
For the 11 patients with complete tracking data from POD 1 to POD 5, the increase in daily steps from POD 1 to POD 5 was statistically significant (P<0.001), as was the increase in the daily duration of PPA (P<0.001). Pairwise comparisons for the number of steps in these patients revealed significant differences between POD 1 and POD 5 (P<0.001), as well as POD 2 and POD 5 (P=0.005). Pairwise comparisons for the duration of PPA revealed significant differences between POD 1 and POD 5 (P<0.001), POD 2 and POD 5 (P=0.005), as well as POD 3 and POD 5 (P=0.01). A repeated measures ANOVA with a Greenhouse-Geisser correction determined for the 38 patients with tracking data from POD 1 to POD 3 that the mean number of steps per day differed significantly between measurements, F(1.45, 53.65)=20.64, P<0.001. In the same way, the duration of PPA showed a significant difference between measurements [F(1.39, 51.38)=17.26, P<0.001]. Bonferroni-adjusted post-hoc analyses revealed significant differences for the number of steps between POD 1 and POD 2 (P=0.02), POD 1 and POD 3 (P<0.001), as well as POD 2 and POD 3 (P<0.001). For the duration of PPA, significant differences obtained by Bonferroni-adjusted post-hoc analyses occurred between POD 1 and POD 2 (P=0.02), POD 1 and POD 3 (P<0.001), as well as POD 2 and POD 3 (P<0.001).
Impact of chest tube removal on physical activity
Patients with complete activity tracking data from the day of chest tube removal and the day after chest tube removal were analyzed as a subgroup (n=20, see Figure 3). The median number of steps and the median duration of PPA approximately tripled between these two measurements; steps: 841 [513–1,884] vs. 2,409 [1,445–2,979]; PPA: 7 [3–15] vs. 20 [11–26] minutes. The mean values for the number of steps (P<0.001) and for the duration of PPA (P=0.001) differed significantly. For the 55 patients with documented pain levels on the numeric rating scale (NRS) from the day of chest tube removal and the day after chest tube removal, a significant decrease in the mean pain level (P=0.001) between both measurements was reported {median pain on NRS: 2 [2–4] vs. 1 [1–2]}. Opioid use also decreased on the day after chest tube removal when compared to the day of chest tube removal (29.1% vs. 36.4%, P=0.13). Figure 4 highlights data on postoperative pain and opioid use from POD 1 to POD 5 for the entire sample.
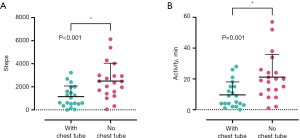
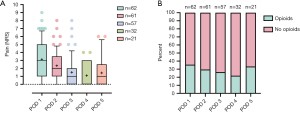
Discussion
We present one of few prospective clinical trials reporting data on PPA after thoracic surgery. The PATHOS study evaluated PPA after thoracoscopic lung resection in a dedicated ERAS-based setting.
One key finding of our study was a substantial, three-fold increase in PPA after removal of the chest tube. Literature already provides ample evidence advocating for early chest tube removal (12-15). Our new data makes it more obvious that in departments where ERAS protocol adhesion is priority, drain management strategy should be equally aggressive to maximize patient benefit.
Another important finding was that both the number of steps and the duration of PPA were rather low during the initial PODs despite an established ERAS-based program. However, we observed a significant increase in both parameters during the hospital stay up to POD 5. We recorded a median of 247 steps on POD 1. To put this step count into perspective, consider the following anecdote: In our 20-bed thoracic surgery unit, a typical patient might leave their bed and walk out of their room at the end of the hallway, to walk towards the patient lounge where they grab a coffee, and then walk back to their bed. Completing this trip twice takes about 280 steps, which is more than most participants in this study did on POD 1. Notably, for 17.7% of study participants, no steps whatsoever were recorded on POD 1. Most surgeons treating patients along ERAS-based pathways do not regard this as sufficient. Furthermore, we can reflect on where we look for our patients when we want to visit them. If the first place we move to is the patient’s room in the unit, the probability is high that mobilization is limited. Based on the unexpected finding that a significant proportion of patients did not achieve early mobilization on POD 1 despite an ERAS protocol, we have comprehensively revised our ERAS pathway in line with the latest research (16,17). A new structured mobility program has been implemented, including a dedicated patient movement course on our ward to further encourage postoperative mobilization.
ERAS concepts have been validated for thoracic surgery and have proven benefits for patients (18,19). In particular, ERAS programs reduce postoperative morbidity and length of hospital stay, notably in patients with lung resections (20,21). However, it remains unclear whether these beneficial effects can be attributed to postoperative mobilization (20,22). While there is no doubt that bed rest beyond short periods of time might be harmful for patients, there is little evidence from which to derive specific postoperative mobilization protocols (6). In a randomized-controlled trial, Jonsson and colleagues found that an in-hospital physiotherapy intervention increased the level of physical activity after surgery for lung cancer (23). However, no increased performance in the 6-minute walk test or in spirometry values was observed in their study, and the authors reported a median number of 659 steps on POD 2 and 805 steps on POD 3. Agostini et al. evaluated physical activity after thoracotomy and lung resection and reported a median number of 170 steps on POD 2 and of 233 steps on POD 3 (24). Finet and colleagues assessed postoperative mobility in patients with minimally invasive thoracic surgery (robotic and thoracoscopic cases) (25). As in the PATHOS trial, their cohort consisted of a mix of lobectomies (66%), segmentectomies (20%), and wedge resections (14%) but reported higher median numbers of steps per day (2,855 steps on POD 1 and up to 3,723 steps on POD 4). One possible explanation is that Finet et al. investigated patients with better fitness than our study (68% of patients classified as ASA 2 vs. 61% of ASA 3 patients in our cohort). However, the authors do not report on preoperative baseline FEV1 or DLCO, which makes the functional assessment of the population challenging.
So, how much postoperative activity and mobilization should we aim for? Regardless of whether ERAS-based protocols were used, prior studies show notable differences in the extent of PPA after lung resections (23-26). For the general population, a goal of 10,000 steps per day is often recommended to promote general health and mental wellbeing (27). An example that such degrees of PPA can be achieved after lung resection has been provided by Esteban et al. (26). In their study, patients underwent minimally invasive lobectomy or bilobectomy and achieved a mean of 8,527 steps on day 0, with 5,738 steps on POD 1 and 10,230 steps on POD 4. However, the authors excluded patients with comorbidities and the mean values for baseline FEV1 and DLCO were 96% and 85% respectively, indicating a selected patient population.
It remains unknown what degree of postoperative activity is necessary to support the essential ERAS effect, that is, to reduce perioperative morbidity. All studies mentioned here had a low complication rate irrespective of the extent of PPA (major morbidity in our sample: n=1 out of 71 patients, 1.4%). However, none of the studies, including ours, were designed or powered to evaluate surgical complications.
This study does have limitations. Our number of patients was small and did not allow for the analysis of all subgroups of interest. The number of patients decreased from POD 1 to POD 5 due to the discharge of patients, which is a common effect in such studies (25). We did not use a research grade accelerometer but a common activity tracker to evaluate PPA and may therefore not have taken all dimensions of physical activity into account. However, non-research grade activity trackers are widely used in research and provide acceptable accuracy (28).
Conclusions
In this single-center prospective observational study, chest tube removal after thoracoscopic lung resection had a major impact on postoperative mobilization (three-fold increase in PPA). However, the observed overall low levels of PPA despite an ERAS setting raise further questions. Larger, comparative studies are needed to determine what type and level of PPA is suitable in today’s era of minimally invasive surgery and ERAS programs and which patients benefit from intensified mobilization to prevent adverse events. Daily steps may not be the only relevant indicator of mobility following lung resection.
Acknowledgments
None.
Footnote
Reporting Checklist: The authors have completed the STROBE reporting checklist. Available at https://jtd.amegroups.com/article/view/10.21037/jtd-2024-2166/rc
Data Sharing Statement: Available at https://jtd.amegroups.com/article/view/10.21037/jtd-2024-2166/dss
Peer Review File: Available at https://jtd.amegroups.com/article/view/10.21037/jtd-2024-2166/prf
Funding: None.
Conflicts of Interest: All authors have completed the ICMJE uniform disclosure form (available at https://jtd.amegroups.com/article/view/10.21037/jtd-2024-2166/coif). The authors have no conflicts of interest to declare.
Ethical Statement: The authors are accountable for all aspects of the work in ensuring that questions related to the accuracy or integrity of any part of the work are appropriately investigated and resolved. The study was conducted in accordance with the Declaration of Helsinki and its subsequent amendments. Institutional review board approval (#2022-16379) was obtained from the ethics commission of the State Chamber of Physicians in Rhineland-Palatinate in Mainz, Germany, on March 21, 2022. Written informed consent was obtained from all patients prior to inclusion.
Open Access Statement: This is an Open Access article distributed in accordance with the Creative Commons Attribution-NonCommercial-NoDerivs 4.0 International License (CC BY-NC-ND 4.0), which permits the non-commercial replication and distribution of the article with the strict proviso that no changes or edits are made and the original work is properly cited (including links to both the formal publication through the relevant DOI and the license). See: https://creativecommons.org/licenses/by-nc-nd/4.0/.
References
- Batchelor TJP, Rasburn NJ, Abdelnour-Berchtold E, et al. Guidelines for enhanced recovery after lung surgery: recommendations of the Enhanced Recovery After Surgery (ERAS®) Society and the European Society of Thoracic Surgeons (ESTS). Eur J Cardiothorac Surg 2019;55:91-115. [Crossref] [PubMed]
- BED REST. thrombosis, and embolism. Lancet 1958;1:465-6. [PubMed]
- Convertino VA. Cardiovascular consequences of bed rest: effect on maximal oxygen uptake. Med Sci Sports Exerc 1997;29:191-6. [Crossref] [PubMed]
- Teba L, Omert LA. Postoperative respiratory insufficiency. Am Fam Physician 1995;51:1473-80. [PubMed]
- Mainini C, Rebelo PF, Bardelli R, et al. Perioperative physical exercise interventions for patients undergoing lung cancer surgery: What is the evidence? SAGE Open Med 2016;4:2050312116673855. [Crossref] [PubMed]
- Castelino T, Fiore JF Jr, Niculiseanu P, et al. The effect of early mobilization protocols on postoperative outcomes following abdominal and thoracic surgery: A systematic review. Surgery 2016;159:991-1003. [Crossref] [PubMed]
- Granger CL, McDonald CF, Berney S, et al. Exercise intervention to improve exercise capacity and health related quality of life for patients with Non-small cell lung cancer: a systematic review. Lung Cancer 2011;72:139-53. [Crossref] [PubMed]
- Smart NJ, White P, Allison AS, et al. Deviation and failure of enhanced recovery after surgery following laparoscopic colorectal surgery: early prediction model. Colorectal Dis 2012;14:e727-34. [Crossref] [PubMed]
- von Elm E, Altman DG, Egger M, et al. The Strengthening the Reporting of Observational Studies in Epidemiology (STROBE) Statement: guidelines for reporting observational studies. Int J Surg 2014;12:1495-9. [Crossref] [PubMed]
- Galata C, Karampinis I, Roessner ED, et al. Risk factors for surgical complications after anatomic lung resections in the era of VATS and ERAS. Thorac Cancer 2021;12:3255-62. [Crossref] [PubMed]
- Seely AJ, Ivanovic J, Threader J, et al. Systematic classification of morbidity and mortality after thoracic surgery. Ann Thorac Surg 2010;90:936-42; discussion 942. [Crossref] [PubMed]
- Cerfolio RJ, Bryant AS. Results of a prospective algorithm to remove chest tubes after pulmonary resection with high output. J Thorac Cardiovasc Surg 2008;135:269-73. [Crossref] [PubMed]
- Bjerregaard LS, Jensen K, Petersen RH, et al. Early chest tube removal after video-assisted thoracic surgery lobectomy with serous fluid production up to 500 ml/day. Eur J Cardiothorac Surg 2014;45:241-6. [Crossref] [PubMed]
- Refai M, Brunelli A, Salati M, et al. The impact of chest tube removal on pain and pulmonary function after pulmonary resection. Eur J Cardiothorac Surg 2012;41:820-2; discussion 823. [Crossref] [PubMed]
- Pfeuty K, Lenot B. Early postoperative day 0 chest tube removal using a digital drainage device protocol after thoracoscopic major pulmonary resection. Interact Cardiovasc Thorac Surg 2020;31:657-63. [Crossref] [PubMed]
- Piler T, Schauer M, Larisch C, et al. Priorities and strategy for the implementation of enhanced recovery after surgery (ERAS) in thoracic surgery. J Thorac Dis 2024;16:4165-73. [Crossref] [PubMed]
- Lu D, Zhou C, Zhang Y, et al. Minimally invasive surgery, precise anesthesia and effective analgesia are crucial for walking within 1 hour after lung surgery. J Thorac Dis 2024;16:5792-801. [Crossref] [PubMed]
- Forster C, Doucet V, Perentes JY, et al. Impact of an enhanced recovery after surgery pathway on thoracoscopic lobectomy outcomes in non-small cell lung cancer patients: a propensity score-matched study. Transl Lung Cancer Res 2021;10:93-103. [Crossref] [PubMed]
- Bang HT, Vy TT, Tuan LQA, et al. Effectiveness of the enhanced recovery after surgery (ERAS) program after lobectomy for lung cancer: a single-center observational study using propensity score matching in Vietnam. J Thorac Dis 2024;16:7686-96. [Crossref] [PubMed]
- Rogers LJ, Bleetman D, Messenger DE, et al. The impact of enhanced recovery after surgery (ERAS) protocol compliance on morbidity from resection for primary lung cancer. J Thorac Cardiovasc Surg 2018;155:1843-52. [Crossref] [PubMed]
- Stuart CM, Dyas AR, Mott NM, et al. Postoperative day of surgery ambulation improves outcomes following lung resection: a multicenter prospective cohort study. J Thorac Dis 2024;16:7300-9. [Crossref] [PubMed]
- Forster C, Doucet V, Perentes JY, et al. Impact of Compliance With Components of an ERAS Pathway on the Outcomes of Anatomic VATS Pulmonary Resections. J Cardiothorac Vasc Anesth 2020;34:1858-66. [Crossref] [PubMed]
- Jonsson M, Hurtig-Wennlöf A, Ahlsson A, et al. In-hospital physiotherapy improves physical activity level after lung cancer surgery: a randomized controlled trial. Physiotherapy 2019;105:434-41. [Crossref] [PubMed]
- Agostini PJ, Naidu B, Rajesh P, et al. Potentially modifiable factors contribute to limitation in physical activity following thoracotomy and lung resection: a prospective observational study. J Cardiothorac Surg 2014;9:128. [Crossref] [PubMed]
- Finet M, Bellicha A, Sage E, et al. Comprehensive assessment of postoperative mobility during the first days after mini-invasive lung surgery: A prospective observational study. J Clin Anesth 2023;86:111048. [Crossref] [PubMed]
- Esteban PA, Hernández N, Novoa NM, et al. Evaluating patients' walking capacity during hospitalization for lung cancer resection. Interact Cardiovasc Thorac Surg 2017;25:268-71. [Crossref] [PubMed]
- Hallam KT, Bilsborough S, de Courten M. "Happy feet": evaluating the benefits of a 100-day 10,000 step challenge on mental health and wellbeing. BMC Psychiatry 2018;18:19. [Crossref] [PubMed]
- Germini F, Noronha N, Borg Debono V, et al. Accuracy and Acceptability of Wrist-Wearable Activity-Tracking Devices: Systematic Review of the Literature. J Med Internet Res 2022;24:e30791. [Crossref] [PubMed]