A bibliometric analysis of the literature published on autophagy, ferroptosis, necroptosis, and pyroptosis in cardiovascular disease from 2009 to 2023
Highlight box
Key findings
• This review completed a comprehensive bibliometric analysis of the literature on programmed cell death (PCD) in cardiovascular disease (CVD) published from 2009 to 2023. The findings highlight significant research trends, influential publications, and emerging hotspots in the fields of autophagy, ferroptosis, necroptosis, and pyroptosis in CVD. The growing impact of machine learning and pharmacological interventions in understanding and targeting these cell death mechanisms emerged as a major theme.
What is known and what is new?
• Autophagy, ferroptosis, necroptosis, and pyroptosis have been implicated in various pathological processes, influencing inflammation, oxidative stress, and cardiomyocyte survival.
• This review mapped the research landscape of PCD in CVD, identifying influential studies, key contributors, and collaborative networks.
What is the implication, and what should change now?
• Greater interdisciplinary collaboration is required to develop innovative interventions that modulate PCD pathways effectively.
• Future research should focus on optimizing pharmacological strategies targeting PCD to improve cardiovascular outcomes.
Introduction
Heart disease is the predominant cause of global mortality (1). Cardiomyocytes are terminally differentiated cells which have limited capacity for differentiation (2), and there is little that can be done once cell death occurs in cardiomyocytes. Abnormal cell death of cardiomyocytes has been implicated in the majority of cardiovascular diseases (CVDs). For instance, the critical event leading to ischemic myocardial injury and heart failure involves the cardiomyocyte death.
Initially, cell death was regarded as an unregulated process. However, a recent research has revealed that cell death is regulated, involving intricate biological processes mediated by numerous molecules and pathways (3). Regulated cell death can be divided into the apoptotic and non-apoptotic types, including autophagy, necroptosis, ferroptosis, pyroptosis, and cuproptosis. Autophagy is a cellular process by which cellular components are captured by autophagosomes and then merged with the lysosome to be degraded (4). Excessive activation of autophagy leads to cell death. Necroptosis is a form of cell death regulated by receptor-interacting protein kinase 1 (RIPK1), receptor-interacting protein kinase 3 (RIPK3), and mixed-lineage kinase domain-like protein (MLKL) (5). Ferroptosis is a type of regulated cell death characterized by iron-dependent lipid peroxidation (6). Glutathione peroxidase 4 (GPX4) and the ferroptosis suppressor protein 1 (FSP1) are key enzymes that suppress ferroptosis by neutralizing lipid peroxides (7). Pyroptosis is a highly regulated cell death characterized by gasdermin D (GSDMD) or gasdermin E (GSDME)-mediated necrosis, with the inflammatory response and typical morphological changes including bubble-like formation, pore formation, and cell swelling (8,9).
The roles of autophagy, ferroptosis, necroptosis, and pyroptosis have emerged across various aspects of cardiovascular research, with each mechanism exhibiting distinct functional relevance depending on the specific disease. The role of autophagy varies significantly across different stages of disease progression and among different cell types (10). Autophagy in myocardial ischemia-reperfusion (I/R) injury acts like a double-edged sword, initially serving as a cellular quality control and survival mechanism, but eventually turning into a harmful process (11-13). Inhibition of necroptosis, ferroptosis and pyroptosis have been shown to confer significant protective effects in various CVDs, including myocardial infarction, atherosclerosis, and abdominal aortic aneurysm (14-16). Necrostatin-1, a necroptosis inhibitor, can potentially mitigate cell loss in the ischemic heart; however, further pre-clinical studies are needed before it could be approved for clinical trials (17,18). Deferiprone (Ferriprox), is a US Food and Drug Administration (FDA)-approved drug used to remove excessive iron from the body of patients with acute myocardial infarction; its mechanism involves the targeting of intramyocardial hemorrhage and suppressing cardiac hypertrophy via the reduction of ferroptosis (19,20). Inhibition of GSDMD significantly reduces cardiomyocyte pyroptosis and I/R-induced myocardial injury (21,22). The data published thus far strongly suggest that autophagy, necroptosis, pyroptosis, and ferroptosis play crucial roles in the development of CVD.
Despite the growing number of studies in this field, a comprehensive and quantitative analysis of the research landscape—including trends, collaborative networks, and emerging hotspots—remains lacking. Bibliometric analysis is a powerful tool that integrates mathematics, statistics, and literature science to assess the impact and evolution of research across disciplines (23). It enables researchers to identify key trends, influential authors and publications, and forecast future directions in each field.
In this study, we conducted a comprehensive bibliometric analysis of publications related to autophagy, necroptosis, ferroptosis, and pyroptosis in CVD from January 1, 2009 to December 31, 2023. Our goal is to uncover research trends, identify leading countries/regions, institutions, authors, journals, and co-citation patterns, and to map the knowledge structure and hotspots in this field. By addressing this gap, our study provides an in-depth overview that may guide future basic and translational research in CVD. We present this article in accordance with the BIBLIO reporting checklist (available at https://jtd.amegroups.com/article/view/10.21037/jtd-2025-682/rc).
Methods
Data collection
The keywords “ferroptosis and cardiovascular”, “necroptosis and cardiovascular”, “pyroptosis and cardiovascular”, and “autophagy and cardiovascular” were each indexed in the Web of Science Core Collection (WoSCC).
On January 2024, we searched the WoSCC for literature published from 2009 to 2023 using the following search formula: “TS = (cardiovascular or heart or circulation) AND TS = (ferroptosis)”, “TS = (cardiovascular or heart or circulation) AND TS = (autophagy)”, “TS = (cardiovascular or heart or circulation) AND TS = (Pyroptosis)”, and “TS = (cardiovascular or heart or circulation) AND TS = (Necroptosis)”. In total, 687 articles related to ferroptosis in CVD, 393 articles related to necroptosis in CVD, 640 articles related to pyroptosis in CVD, and 6,426 articles related to autophagy in CVD were retrieved, and the records were exported with all references, saved as plain text files, and stored in saved recs_text format.
Only peer-reviewed journal articles were included, while grey literature such as preprints, conference abstracts, and unpublished studies were excluded. Citation analysis, co-authorship networks, and journal co-citation patterns were used to identify the influential authors, institutions, and research hotspots. Only studies published in the English language were included in the analysis. No additional contact with study authors or trial registries was required.
To ensure the accuracy and consistency of the review, the selected papers were independently assessed by H.Z., Y.Z. and T.L. Each paper was evaluated based on predefined criteria, including relevance to the research questions, study quality, and methodological rigor. The assessment was guided by a standardized checklist to minimize bias and ensure a systematic evaluation (Figure S1).
Statistical analysis
The “bibliometrix” R package (The R Foundation for Statistical Computing, Vienna, Austria) was used to download and analyze the general information of the literature, including year of publication, country, organization, journal, and author. Subsequently, VOSviewer software version 1.6.18 (Leiden University’s Centre for Science and Technology Studies, Leiden, the Netherlands) was employed to conduct bibliometric and visualization analysis. The review included co-authorship, co-occurrence, citation, bibliographic coupling, and co-citation analyses. For co-authorship analysis, the relevance of papers was determined according to the number of co-authored documents. For co-occurrence analysis, the relevance of papers was determined according to the number of documents they co-occurred in. For citation analysis, the relevance was determined according to the number of citations. For bibliographic coupling analysis, the relevance of papers was determined according to the number of shared citations of the analyzed papers. For co-citation analysis, the relevance of papers was determined by the number of times two appeared papers in the same document. Ranking was based on a counting method, and the association strength was normalized in VOSviewer software. Microsoft Office Excel 2019 (Microsoft Corp., Redmond, WA, USA) was used to analyze the number of publications per year.
Results
The annual trends of publications
The quantity of publications during a period indicates the prevailing research interests within the field. The number of publications on autophagy in CVD, ferroptosis in CVD, necroptosis in CVD, and pyroptosis in CVD increased annually overall. For autophagy, the volume of literature increased steadily from 2009 to 2021 but remained stagnant from 2021 to 2023. The volume of literature on necroptosis CVD increased steadily year by year from 2013 to 2023. For ferroptosis in CVD, the volume of literature grew rapidly year to year starting in 2015. Similar to ferroptosis in CVD, the number of publications on pyroptosis in CVD increased gradually beginning in 2012 (Figure 1).
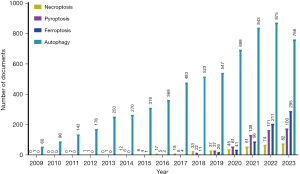
Distribution of countries/regions and organizations
For autophagy in CVD, 4,891 institutions from 90 different countries/regions published 6,426 articles. In the field of necroptosis CVD, 393 articles were published from 616 organizations in 53 countries/regions. For pyroptosis in CVD, 754 institutions from 48 various countries/regions contributed 640 publications. Ferroptosis in CVD, 687 articles were published from 827 institutions in 49 countries/regions. Both China and the United States ranked as top countries in the number of articles and total citations related to autophagy, necroptosis, pyroptosis, and ferroptosis in CVD. All the top 10 institutions, except for Comenius University Bratislava, University of Washington Seattle, Slovak Academy of Sciences, Chiang Mai University, Virginia Commonwealth University, and the University of Nicosia, were from China. In the fields of necroptosis in CVD, Comenius University Bratislava ranked first in the number of articles. Meanwhile, Harbin Medical University achieved the top rank in terms of the number of publications on pyroptosis in CVD factor (CVDF). Fudan University ranked the first in the number of publications in the field of autophagy in CVD. For ferroptosis in CVD, Zhejiang University contributed to the largest number of publications (Table 1).
Table 1
Type | Rank | Country | Organization | |||||||
---|---|---|---|---|---|---|---|---|---|---|
Country | Avg.pub.year | Documents | Citations | Organization | Avg.pub.year | Documents | Citations | |||
Necroptosis | 1 | China | 2021 | 199 | 6,056 | Comenius University Bratislava | 2019 | 22 | 697 | |
2 | USA | 2019 | 96 | 7,041 | Nantong University | 2020 | 15 | 265 | ||
3 | Slovakia | 2019 | 23 | 3,730 | Fudan University | 2021 | 15 | 330 | ||
4 | Germany | 2018 | 25 | 697 | Central South University | 2020 | 12 | 233 | ||
5 | Canada | 2020 | 21 | 742 | University of Washington Seattle | 2020 | 11 | 769 | ||
6 | England | 2022 | 18 | 468 | Slovak Academy of Sciences | 2021 | 10 | 207 | ||
7 | Japan | 2020 | 14 | 570 | Chiang Mai University | 2022 | 9 | 97 | ||
8 | India | 2021 | 10 | 213 | Nanchang University | 2021 | 9 | 311 | ||
9 | Thailand | 2022 | 10 | 229 | Chinese Academy of Medical Sciences | 2022 | 9 | 716 | ||
10 | Russia | 2020 | 9 | 471 | Capital Medical University | 2022 | 8 | 83 | ||
Pyroptosis | 1 | China | 2022 | 482 | 10,898 | Harbin Medical University | 2021 | 39 | 1,618 | |
2 | USA | 2019 | 99 | 8,641 | Fudan University | 2021 | 22 | 682 | ||
3 | England | 2020 | 19 | 722 | Sun Yat Sen University | 2021 | 21 | 788 | ||
4 | Germany | 2020 | 17 | 1,680 | Nanjing Medical University | 2022 | 20 | 432 | ||
5 | Italy | 2022 | 13 | 466 | Wenzhou Medical University | 2021 | 18 | 384 | ||
6 | India | 2022 | 11 | 73 | Zhejiang University | 2022 | 18 | 140 | ||
7 | Russia | 2022 | 10 | 259 | University of South China | 2022 | 18 | 551 | ||
8 | Spain | 2021 | 10 | 413 | Chinese Academy of Medical Sciences | 2021 | 17 | 331 | ||
9 | Japan | 2020 | 10 | 671 | Shanghai Jiao Tong University | 2022 | 16 | 233 | ||
10 | Canada | 2021 | 8 | 321 | Wuhan university | 2022 | 15 | 539 | ||
Ferroptosis | 1 | China | 2022 | 522 | 12,886 | Zhejiang University | 2022 | 28 | 2,092 | |
2 | USA | 2021 | 99 | 8,550 | Wuhan university | 2022 | 26 | 678 | ||
3 | Japan | 2021 | 22 | 1,204 | Fudan University | 2022 | 23 | 590 | ||
4 | Germany | 2020 | 20 | 2,494 | Central South University | 2022 | 22 | 704 | ||
5 | Canada | 2021 | 14 | 404 | Nanjing Medical University | 2022 | 21 | 250 | ||
6 | Italy | 2022 | 10 | 516 | Southern Medical University | 2022 | 19 | 221 | ||
7 | Russia | 2022 | 10 | 323 | Shanghai Jiao Tong University | 2022 | 18 | 1,373 | ||
8 | India | 2022 | 9 | 259 | Harbin Medical University | 2022 | 17 | 177 | ||
9 | Korea | 2021 | 9 | 225 | Nanchang University | 2022 | 17 | 182 | ||
10 | Iran | 2022 | 9 | 39 | Huazhong University of Science and Technology | 2022 | 16 | 209 | ||
Autophagy | 1 | China | 2020 | 3099 | 73,121 | Fudan University | 2019 | 191 | 5,358 | |
2 | USA | 2017 | 1833 | 97,772 | Shanghai Jiao Tong University | 2019 | 131 | 4,644 | ||
3 | Italy | 2019 | 329 | 16,139 | Air Force Military Medical University | 2018 | 125 | 5,489 | ||
4 | Japan | 2017 | 294 | 16,195 | Shandong University | 2019 | 121 | 3,232 | ||
5 | Germany | 2017 | 276 | 14,707 | Capital Medical University | 2019 | 118 | 2,980 | ||
6 | England | 2018 | 214 | 15,389 | University of Wyoming | 2014 | 103 | 5,240 | ||
7 | Canada | 2017 | 203 | 10,465 | Nanjing Medical University | 2019 | 103 | 2,353 | ||
8 | France | 2019 | 164 | 8,901 | Zhejiang University | 2021 | 101 | 3,251 | ||
9 | India | 2020 | 145 | 5,231 | Wuhan university | 2020 | 100 | 2,135 | ||
10 | Spain | 2019 | 145 | 3,729 | Huazhong University of Science and Technology | 2020 | 95 | 2,149 |
Avg.pub.year, average publication year.
Cooperation between countries/regions, as indicated by co-authorship (Figure S2), citation (Figure S3), and bibliographic coupling analyses (Figure S4), was mainly observed between China and the United States, with limited cooperation evident between other nations. The collaboration between institutions, as indicated by co-authorship (Figure S5), citation (Figure S6), and bibliographic coupling analyses (Figure S7), was more complex. Large institutions had a diversity of connections with other organizations; however, some organizations remain isolated.
Co-authorship, citation, bibliographic coupling, and co-citation analyses for authors
In the fields of necroptosis in CVD, pyroptosis in CVD, ferroptosis in CVD, and autophagy in CVD, there were 2,435, 4,125, 4,432, and 31,253 authors involved in the publications, respectively. In the field of necroptosis in CVD, Adameova A published the highest number of papers (n=22), followed by Adrian A (14) and Zhang W (12). In the field of pyroptosis in CVD, Ge J and Ye B ranked first in the number of publications, each with 8 papers published. In the field of autophagy in CVD and ferroptosis in CVD, Ren J ranked first with 120 papers published and 10 papers published, respectively (Table 2, Figures S8,S9, Figure 2). Co-cited authors are authors who are cited in the same paper, forming a co-citation relationship.
Table 2
Type | Rank | The top 10 authors | The top 10 Co-cited author | |||||
---|---|---|---|---|---|---|---|---|
Author | Avg.pub.year | Documents | Citations | Co-cited author | Citations | |||
Necroptosis | 1 | Adameova, Adriana | 2019 | 22 | 625 | Degterev, A | 157 | |
2 | Szobi, Adrian | 2017 | 14 | 287 | Linkermann, A | 138 | ||
3 | Zhang, Wei | 2021 | 12 | 106 | Zhang, T | 134 | ||
4 | Chattipakorn, Nipon | 2021 | 9 | 97 | Galluzzi, L | 128 | ||
5 | Chattipakorn, Siriporn C | 2021 | 9 | 97 | He, SD | 99 | ||
6 | Maneechote, Chayodom | 2022 | 8 | 65 | Newton, K | 94 | ||
7 | Zhang, Jingjing | 2018 | 7 | 7 | Zhou, H | 89 | ||
8 | Qian, Jianan | 2022 | 7 | 7 | Cho, Y | 81 | ||
9 | Arunsak, Busarin | 2023 | 7 | 44 | Luedde, M | 81 | ||
10 | Horvath, Csaba | 2022 | 7 | 64 | Sun, LM | 80 | ||
Pyroptosis | 1 | Ge, Junbo | 2021 | 8 | 239 | Shi, JJ | 273 | |
2 | Ye, Bozhi | 2022 | 8 | 211 | Zhang, Y | 179 | ||
3 | Dai, Shanshan | 2022 | 7 | 210 | Toldo, S | 175 | ||
4 | Mishra, Paras K. | 2019 | 7 | 225 | Kayagaki, N | 135 | ||
5 | Wang, Hong | 2021 | 7 | 403 | Liu, X | 123 | ||
6 | Yang, Wei | 2021 | 7 | 149 | Lamkanfi, M | 118 | ||
7 | Zhang, Jing | 2022 | 7 | 165 | Wang, Y | 116 | ||
8 | Huang, Weijian | 2022 | 6 | 144 | Liu, Y | 107 | ||
9 | Abbate, Antonio | 2021 | 6 | 944 | Zeng, ZL | 107 | ||
10 | Jiang, Xiaohua | 2020 | 6 | 402 | Li, X | 101 | ||
Ferroptosis | 1 | Ren, Jun | 2022 | 10 | 204 | Dixon, SJ | 542 | |
2 | Matsui, Takashi | 2020 | 9 | 463 | Fang, XX | 438 | ||
3 | Li, Wei | 2022 | 9 | 239 | Yang, WS | 420 | ||
4 | Chattipakorn, Nipon | 2022 | 9 | 88 | Stockwell, BR | 288 | ||
5 | Higa, Jason K. | 2019 | 8 | 443 | Gao, MH | 281 | ||
6 | Min, Junxia | 2022 | 7 | 1,908 | Doll, S | 250 | ||
7 | Wang, Fudi | 2022 | 7 | 1,908 | Angeli, JPF | 210 | ||
8 | Peng, Jun | 2022 | 7 | 356 | Chen, X | 202 | ||
9 | Liu, Ying | 2023 | 7 | 155 | Wang, Y | 152 | ||
10 | Chattipakorn, Nipon | 2022 | 7 | 81 | Li, N | 146 | ||
Autophagy | 1 | Ren, Jun | 2016 | 120 | 5,368 | Mizushima, N | 1,579 | |
2 | Sadoshima, Junichi | 2018 | 106 | 7,364 | Levine, B | 1,052 | ||
3 | Zhang, Yingmei | 2017 | 57 | 3,027 | Klionsky, DJ | 905 | ||
4 | Gottlieb, Roberta A. | 2014 | 53 | 3,511 | Sciarretta, S | 877 | ||
5 | Hill, Joseph A. | 2013 | 48 | 3,832 | Matsui, Y | 775 | ||
6 | Gustafsson, Asa B. | 2014 | 46 | 3,777 | Nakai, A | 685 | ||
7 | Sciarretta, Sebastiano | 2015 | 40 | 3,874 | Wang, Y | 680 | ||
8 | Zhai, Peiyong | 2015 | 39 | 3,295 | Zhang, Y | 637 | ||
9 | Kroemer, Guido | 2019 | 38 | 2,577 | Kim, J | 607 | ||
10 | Lavandero, Sergio | 2016 | 35 | 2,190 | Chen, Y | 527 |
Avg.pub.year, average publication year.

In the fields of necroptosis in CVD, pyroptosis in CVD, ferroptosis in CVD, and autophagy in CVD, there were 16,024, 24,681, 26,648, and 138,237 co-cited authors involved in the publication of literature, respectively (Table 2, Figure 3). In the field of necroptosis in CVD, Degterev A was the most cited author (157 times), followed by Linkermann A (138 times) and Zhang T (134 times). In the field of pyroptosis in CVD, Shi JJ ranked first with 273 citations, followed by Zhang Y (179 times) and Toldo S (175 times). In the field of ferroptosis in CVD, Dixon SJ had the most citations (542 times), followed by Fang XX (438 times) and Yang WS (420 times). Mizushima N was cited 1,579 times and was ranked first in the field of autophagy in CVD, followed by Levine B (1,052 times) and Klionsky DJ (905 times).
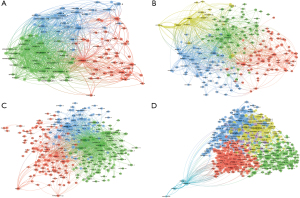
Citation, bibliographic, and co-citation analyses for journals
In the field of necroptosis in CVD, 220 journals published 393 articles. In the field of pyroptosis in CVD, 290 journals published 640 articles. In the field of ferroptosis in CVD, 297 journals published 687 papers. In the field of autophagy in CVD, 1,245 journals published 6,426 papers. The International Journal of Molecular Sciences published 13 papers, placing it first place in the field of necroptosis in CVD, followed by Frontiers in Cardiovascular Medicine (12 articles) and Frontiers in Pharmacology (10 articles). In the field of pyroptosis in CVD, 24 papers were published in Frontiers in Cardiovascular Medicine which had the highest number of articles, followed by Oxidative Medicine and Cellular Longevity (17 articles) and Cell Death & Disease (16 articles). In the field of ferroptosis in CVD, Frontiers in Cardiovascular Medicine had the highest number of publications (29 articles), followed by Frontiers in Pharmacology (23 articles) and Free Radical Biology and Medicine (19 articles). In the field of autophagy in CVD, the International Journal of Molecular Sciences had 136 papers, placing it first, followed by Circulation (134 articles) and the Journal of Molecular and Cellular Cardiology (128 articles). In the field of necroptosis in CVD, ferroptosis in CVD, and autophagy in CVD, Circulation (Q1) had the highest impact factor (IF; 39.918). In the field of pyroptosis in CVD, Cell Death & Disease (Q2) had the highest IF (12.100) (Table 3 and Figure 4, Figure S10).
Table 3
Type | Rank | The top 10 journal | The top 10 co-cited journal | ||||||||
---|---|---|---|---|---|---|---|---|---|---|---|
Journal | Documents | Citation | IF [2023] | JCR | Co-cited journal | Citation | IF [2023] | JCR | |||
Necroptosis | 1 | International Journal of Molecular Sciences | 13 | 165 | 6.208 | Q2 | Cell | 858 | 64.500 | Q1 | |
2 | Frontiers in Cardiovascular Medicine | 12 | 164 | 3.600 | Q2 | Nature | 762 | 63.580 | Q1 | ||
3 | Frontiers in Pharmacology | 10 | 277 | 4.225 | Q1 | Cell Death & Difference | 708 | 12.067 | Q2 | ||
4 | Oxidative Medicine and Cellular Longevity | 8 | 92 | 7.310 | Q2 | Proceedings of the National Academy of Sciences of the United States of America | 689 | 12.779 | Q1 | ||
5 | Journal of Cellular and Molecular Medicine | 7 | 189 | 4.302 | Q2 | Circulation | 664 | 39.918 | Q1 | ||
6 | Journal of Molecular and Cellular Cardiology | 7 | 258 | 5.700 | Q2 | Circulation Research | 632 | 20.100 | Q1 | ||
7 | Biomedicine & Pharmacotherapy | 6 | 62 | 7.419 | Q1 | Journal of Biological Chemistry | 605 | 5.486 | Q2 | ||
8 | Circulation | 6 | 174 | 39.918 | Q1 | Cell Death & Disease | 510 | 9.685 | Q2 | ||
9 | International Journal of Molecular Medicine | 6 | 116 | 3.098 | Q3 | Cardiovascular Research | 407 | 13.081 | Q1 | ||
10 | Molecular and Cellular Biochemistry | 6 | 111 | 3.842 | Q4 | Journal of Molecular And Cellular Cardiology | 403 | 5.763 | Q2 | ||
Pyroptosis | 1 | Frontiers in Cardiovascular Medicine | 24 | 197 | 3.600 | Q2 | Nature | 1,524 | 63.580 | Q1 | |
2 | Oxidative Medicine and Cellular Longevity | 17 | 376 | 7.310 | Q2 | Circulation | 1,004 | 39.918 | Q1 | ||
3 | Cell Death & Disease | 16 | 1,295 | 12.100 | Q2 | Circulation Research | 935 | 20.100 | Q1 | ||
4 | Frontiers In Pharmacology | 16 | 313 | 5.988 | Q2 | Proceedings of the National Academy of Sciences of The United States of America | 824 | 12.779 | Q1 | ||
5 | Biomedicine & Pharmacotherapy | 15 | 176 | 7.419 | Q1 | Cell | 803 | 64.500 | Q1 | ||
6 | Frontiers in Cell and Developmental Biology | 15 | 267 | 6.081 | Q1 | Cell Death & Disease | 744 | 9.685 | Q2 | ||
7 | International Journal of Molecular Sciences | 15 | 141 | 6.208 | Q1 | Journal of Biological Chemistry | 721 | 5.486 | Q2 | ||
8 | Frontiers in Immunology | 14 | 713 | 7.561 | Q1 | PLoS One | 628 | 3.752 | Q2 | ||
9 | International Immunopharmacology | 14 | 227 | 5.714 | Q2 | Biochemical and Biophysical Research Communications | 543 | 3.322 | Q3 | ||
10 | Biochemical and Biophysical Research Communications | 11 | 231 | 3.300 | Q3 | Oxidative Medicine and Cellular Longevity | 530 | 7.310 | Q2 | ||
Ferroptosis | 1 | Frontiers in Cardiovascular Medicine | 29 | 300 | 3.600 | Q2 | Cell | 1,480 | 64.500 | Q1 | |
2 | Frontiers in Pharmacology | 23 | 479 | 5.988 | Q2 | Free Radical Biology and Medicine | 1,306 | 8.101 | Q2 | ||
3 | Free Radical Biology and Medicine | 19 | 512 | 8.101 | Q2 | Nature | 1,223 | 63.580 | Q1 | ||
4 | Biomedicine & Pharmacotherapy | 17 | 233 | 7.419 | Q2 | Proceedings of the National Academy of Sciences of The United States of America | 1,127 | 12.779 | Q1 | ||
5 | Oxidative Medicine and Cellular Longevity | 17 | 351 | 7.310 | Q2 | Journal of Biological Chemistry | 882 | 5.486 | Q1 | ||
6 | Frontiers in Cell and Developmental Biology | 16 | 341 | 5.500 | Q3 | Cell Death & Disease | 855 | 9.685 | Q2 | ||
7 | International Journal of Molecular Sciences | 14 | 319 | 6.208 | Q1 | Circulation | 842 | 39.918 | Q1 | ||
8 | Cells | 11 | 138 | 7.666 | Q2 | Circulation Research | 835 | 20.100 | Q1 | ||
9 | Life Sciences | 9 | 556 | 6.780 | Q2 | Biochemical and Biophysical Research Communications | 820 | 3.322 | Q3 | ||
10 | Antioxidants | 8 | 243 | 7.675 | Q2 | Cell Death & Difference | 782 | 12.067 | Q2 | ||
Autophagy | 1 | International Journal of Molecular Sciences | 136 | 2,564 | 6.208 | Q1 | Journal of Biological Chemistry | 12,012 | 5.486 | Q2 | |
2 | Circulation | 134 | 3,995 | 39.918 | Q1 | Circulation Research | 11,966 | 20.100 | Q1 | ||
3 | Journal of Molecular and Cellular Cardiology | 128 | 6,347 | 5.763 | Q2 | Autophagy | 10,239 | 13.300 | Q1 | ||
4 | Frontiers in Pharmacology | 117 | 1,878 | 5.988 | Q2 | Circulation | 9,868 | 39.918 | Q1 | ||
5 | Circulation Research | 114 | 12,149 | 20.100 | Q1 | Nature | 8,510 | 63.580 | Q1 | ||
6 | PLoS One | 109 | 4,270 | 3.752 | Q2 | Proceedings of the National Academy of Sciences of the United States of America | 8,026 | 12.779 | Q1 | ||
7 | Autophagy | 106 | 6,503 | 13.300 | Q1 | Cell | 7,224 | 11.091 | Q1 | ||
8 | Oxidative Medicine and Cellular Longevity | 95 | 3,609 | 7.310 | Q2 | PLoS One | 7,056 | 3.752 | Q2 | ||
9 | Frontiers in Cardiovascular Medicine | 94 | 987 | 3.600 | Q2 | Journal of Molecular and Cellular Cardiology | 5,693 | 5.763 | Q2 | ||
10 | Biochemical and Biophysical Research Communications | 81 | 2,142 | 3.300 | Q3 | Journal of Clinical Investigation | 5,624 | 19.456 | Q1 |
IF, impact factor; JCR, Journal Citation Reports.
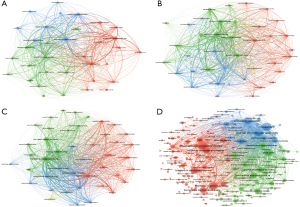
Journal co-citation analysis is a valuable tool for examining which journals are frequently cited together. The results of our co-citation analysis indicated that in the field of necroptosis in CVD, Cell and Nature were the two most cited journals. In the field of pyroptosis in CVD, Nature and Circulation were two most cited journals (over 1,000 citations). In the field of ferroptosis in CVD, Free Radical Biology and Medicine and Nature were two most cited journals (over 1,000 citations). In the field of autophagy in CVD, the Journal of Biological Chemistry and Circulation Research were the two most cited journals (over 1,000 citations) (Table 3, Figure 5).
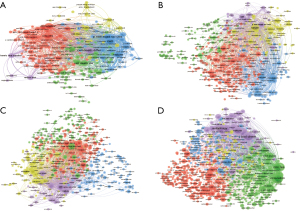
Citations and co-citations
In the field of necroptosis in CVD, the article “CaMKII is a RIP3 substrate mediating ischemia- and oxidative stress-induced myocardial necroptosis” was the most cited paper (514 citations) (24). For pyroptosis in CVD, the article “Pyroptosis: host cell death and inflammation” was the most cited paper (2,105 citations) (25). In the field of ferroptosis in CVD, the article “Ferroptosis as a target for protection against cardiomyopathy” published in Cell Death and Differentiation was cited 1,959 times (26), placing it first in the field. The most cited publication in autophagy in CVD was the article “Cardioprotection and lifespan extension by the natural polyamine spermidine” published in Nature Medicine (27) (Table 4, Figures 6,7).
Table 4
Type | Rank | Literature | Title | DOI | Source | IF/JCR | Citations |
---|---|---|---|---|---|---|---|
Necroptosis | 1 | Zhang T [2016] | CaMKII is a RIP3 substrate mediating ischemia- and oxidative stress-induced myocardial necroptosis (24) | 10.1038/nm.4017 | Nature Medicine | 82.9/Q1 | 514 |
2 | Del Re DP [2019] | Fundamental Mechanisms of Regulated Cell Death and Implications for Heart Disease (28) | 10.1152/physrev.00022.2018 | Physiological Reviews | 29.9/Q1 | 471 | |
3 | Zhu P [2018] | Ripk3 promotes ER stress-induced necroptosis in cardiac IR injury: A mechanism involving calcium overload/XO/ROS/mPTP pathway (29) | 10.1016/j.redox.2018.02.019 | Redox Biology | 11.4/Q1 | 283 | |
4 | Luedde M [2014] | RIP3, a kinase promoting necroptotic cell death, mediates adverse remodelling after myocardial infarction (30) | 10.1093/cvr/cvu146 | Cardiovascular Research | 7.0/Q1 | 228 | |
5 | Qin D [2016] | MicroRNA-223-5p and -3p Cooperatively Suppress Necroptosis in Ischemic/Reperfused Hearts (31) | 10.1074/jbc.M116.732735 | Journal of Biological Chemistry | 4.0/Q2 | 105 | |
6 | Koshinuma S [2014] | Combination of necroptosis and apoptosis inhibition enhances cardioprotection against myocardial ischemia-reperfusion injury (32) | 10.1007/s00540-013-1716-3 | Journal of Anesthesia | 2.8/Q2 | 96 | |
7 | Zhe-Wei S [2018] | The Role of Necroptosis in Cardiovascular Disease (33) | 10.3389/fphar.2018.00721 | Frontiers in Pharmacology | 4.4/Q1 | 82 | |
8 | Guo X [2017] | Cardioprotective Role of Tumor Necrosis Factor Receptor-Associated Factor 2 by Suppressing Apoptosis and Necroptosis (34) | 10.1161/CIRCULATIONAHA.116.026240 | Circulation | 35.5/Q1 | 66 | |
9 | Adameova A [2016] | Necroptotic cell death in failing heart: relevance and proposed mechanisms (22) | 10.1007/s10741-016-9537-8 | Heart Failure Reviews | 4.5/Q1 | 64 | |
10 | Szobi A [2017] | Analysis of necroptotic proteins in failing human hearts (35) | 10.1186/s12967-017-1189-5 | Journal of Translational Medicine | 6.1/Q1 | 55 | |
Pyroptosis | 1 | Bergsbaken T [2009] | Pyroptosis: host cell death and inflammation (25) | 10.1038/nrmicro2070 | Nature Reviews Microbiology | 69.2/Q1 | 2,105 |
2 | Del Re DP [2019] | Fundamental Mechanisms of Regulated Cell Death and Implications for Heart Disease (28) | 10.1152/physrev.00022.2018 | Physiological Reviews | 33.6/Q1 | 471 | |
3 | Mezzaroma E [2011] | The inflammasome promotes adverse cardiac remodeling following acute myocardial infarction in the mouse (36) | 10.1073/pnas.1108586108 | Proceedings of the National Academy of Sciences of the United States of America | 11.2/Q1 | 458 | |
4 | Wu X [2018] | Nicotine promotes atherosclerosis via ROS-NLRP3-mediated endothelial cell pyroptosis (37) | 10.1038/s41419-017-0257-3 | Cell Death & Disease | 9.0/Q1 | 343 | |
5 | Zhaolin Z [2019] | Role of pyroptosis in cardiovascular disease (16) | 10.1111/cpr.12563 | Cell Proliferation | 8.5/Q1 | 241 | |
6 | Toldo S [2018] | Inflammasome, pyroptosis, and cytokines in myocardial ischemia-reperfusion injury (38) | 10.1152/ajpheart.00158.2018 | American Journal of Physiology-Heart and Circulatory Physiology | 4.1/Q1 | 221 | |
7 | Li X [2014] | MicroRNA-30d regulates cardiomyocyte pyroptosis by directly targeting foxo3a in diabetic cardiomyopathy (39) | 10.1038/cddis.2014.430 | Cell Death & Disease | 9.0/Q1 | 220 | |
8 | Zeng C [2019] | Role of Pyroptosis in Cardiovascular Diseases and its Therapeutic Implications (40) | 10.7150/ijbs.33568 | International Journal of Biological Sciences | 8.2/Q1 | 167 | |
9 | Jia C [2019] | Role of pyroptosis in cardiovascular diseases (41) | 10.1016/j.intimp.2018.12.028 | International Immunopharmacology | 4.8/Q1 | 148 | |
10 | Shi H [2021] | GSDMD-Mediated Cardiomyocyte Pyroptosis Promotes Myocardial I/R Injury (21) | 10.1161/CIRCRESAHA.120.318629 | Circulation Research | 20.1/Q1 | 118 | |
Ferroptosis | 1 | Xie Y [2016] | Ferroptosis: process and function (42) | 10.1038/cdd.2015.158 | Cell Death and Differentiation | 12.4/Q1 | 1,959 |
2 | Gao M [2015] | Glutaminolysis and Transferrin Regulate Ferroptosis (43) | 10.1016/j.molcel.2015.06.011 | Molecules and Cells | 4.3/Q3 | 1,111 | |
3 | Fang X [2019] | Ferroptosis as a target for protection against cardiomyopathy (26) | 10.1073/pnas.1821022116 | Proceedings of the National Academy of Sciences of the United States of America | 11.2/Q1 | 1,019 | |
4 | Dodson M [2019] | NRF2 plays a critical role in mitigating lipid peroxidation and ferroptosis (44) | 10.1016/j.redox.2019.101107 | Redox Biology | 11.4/Q1 | 795 | |
5 | Del Re DP [2019] | Fundamental Mechanisms of Regulated Cell Death and Implications for Heart Disease (28) | 10.1152/physrev.00022.2018 | Physiological Reviews | 33.6/Q1 | 471 | |
6 | Fang X [2020] | Loss of Cardiac Ferritin H Facilitates Cardiomyopathy via Slc7a11-Mediated Ferroptosis (45) | 10.1161/CIRCRESAHA.120.316509 | Circulation Research | 20.1/Q1 | 329 | |
7 | Li W [2019] | Ferroptotic cell death and TLR4/Trif signaling initiate neutrophil recruitment after heart transplantation (46) | 10.1172/JCI126428 | Journal of Clinical Investigation | 15.9/Q1 | 263 | |
8 | Baba Y [2018] | Protective effects of the mechanistic target of rapamycin against excess iron and ferroptosis in cardiomyocytes (2) | 10.1152/ajpheart.00452.2017 | American Journal of Physiology-Heart and Circulatory Physiology | 4.1/Q1 | 216 | |
9 | Park TJ [2019] | Quantitative proteomic analyses reveal that GPX4 downregulation during myocardial infarction contributes to ferroptosis in cardiomyocytes (47) | 10.1038/s41419-019-2061-8 | Cell Death & Disease | 9.0/Q1 | 177 | |
10 | Liu B [2018] | Puerarin protects against heart failure induced by pressure overload through mitigation of ferroptosis (48) | 10.1016/j.bbrc.2018.02.061 | Biochemical and Biophysical Research Communications | 2.5/Q3 | 138 | |
Autophagy | 1 | Eisenberg T [2016] | Cardioprotection and lifespan extension by the natural polyamine spermidine (27) | 10.1038/nm.4222 | Nature Medicine | 82.9/Q1 | 681 |
2 | Bravo-San Pedro JM [2017] | Autophagy and Mitophagy in Cardiovascular Disease (49) | 10.1161/CIRCRESAHA.117.311082 | Circulation Research | 20.1/Q1 | 479 | |
3 | Ikeda Y [2015] | Endogenous Drp1 mediates mitochondrial autophagy and protects the heart against energy stress (50) | 10.1161/CIRCRESAHA.116.303356 | Circulation Research | 20.1/Q1 | 411 | |
4 | Xie Z [2011] | Improvement of cardiac functions by chronic metformin treatment is associated with enhanced cardiac autophagy in diabetic OVE26 mice (51) | 10.2337/db10-0351 | Diabetes | 6.2/Q1 | 401 | |
5 | Ma X [2012] | Impaired autophagosome clearance contributes to cardiomyocyte death in ischemia/reperfusion injury (52) | 10.1161/CIRCULATIONAHA.111.041814 | Circulation | 35.5/Q1 | 379 | |
6 | Kubli DA [2013] | Parkin protein deficiency exacerbates cardiac injury and reduces survival following myocardial infarction (53) | 10.1074/jbc.M112.411363 | Journal of Biological Chemistry | 4.0/Q2 | 352 | |
7 | Taneike M [2010] | Inhibition of autophagy in the heart induces age-related cardiomyopathy (54) | 10.4161/auto.6.5.11947 | Autophagy | 14.6/Q1 | 337 | |
8 | Sciarretta S [2018] | The Role of Autophagy in the Heart (55) | 10.1146/annurev-physiol-021317-121427 | Annual Review of Physiology | 15.7/Q1 | 317 | |
9 | Nishida K [2009] | The role of autophagy in the heart (56) | 10.1038/cdd.2008.163 | Cell Death and Differentiation | 12.4/Q1 | 316 | |
10 | Gustafsson AB [2009] | Autophagy in ischemic heart disease (57) | 10.1161/CIRCRESAHA.108.187427 | Circulation Research | 20.1/Q1 | 314 |
IF, impact factor; JCR, Journal Citation Reports.
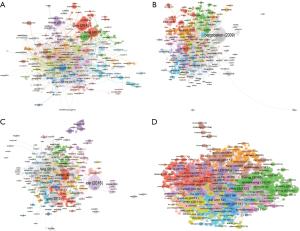
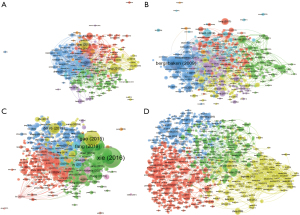
Table 5 and Figure 8 list the top 10 references with the most co-citations in the field of necroptosis in CVD, pyroptosis in CVD, ferroptosis in CVD, and autophagy in CVD. As shown in Table 5, the paper with most co-citations in the field of necroptosis in CVD was by Zhang et al. who identified CaMKII as a novel substrate of RIP3, outlining a RIP3-CaMKII-mPTP pathway involved in myocardial necroptosis (24). The article with the most co-citations in the field of pyroptosis in CVD found that GSDMD plays a critical role in caspase-11 and caspase-1-mediated pyroptosis in mouse bone marrow macrophages. In the field of ferroptosis in CVD, the article with the most co-citations was by Dixon et al. (73), who identified ferrostatin-1 as a strong inhibitor of ferroptosis in cancer cells and of glutamate-induced cell death in organotypic rat brain slices, indicating potential similarities between these two processes. In the field of autophagy in CVD, the article with the most co-citations was Nakai et al. (78) who found that autophagy in the heart under normal conditions acts as a homeostatic mechanism to maintain the size of cardiomyocytes and overall cardiac structure and function; additionally, they found that increased autophagy in failing hearts serves as an adaptive response to protect cells from the stress caused by hemodynamic changes.
Table 5
Type | Rank | Reference | Co-citations | Year |
---|---|---|---|---|
Necroptosis | 1 | Zhang T, 2016, Nat Med, V22, p175, doi: 10.1038/nm.4017 (24) | 117 | 2016 |
2 | Luedde M, 2014, Cardiovasc Res, V103, p206, doi: 10.1093/cvr/cvu146 (30) | 81 | 2014 | |
3 | Sun L, 2012, Cell, V148, p213, doi: 10.1016/j.cell.2011.11.031 (58) | 76 | 2012 | |
4 | Cho Y, 2009, Cell, V137, p1112, doi: 10.1016/j.cell.2009.05.037 (59) | 75 | 2009 | |
5 | Degterev A, 2005, Nat Chem Biol, V1, p112, doi: 10.1038/nchembio711 (60) | 72 | 2005 | |
6 | He S, 2009, Cell, V137, p1100, doi: 10.1016/j.cell.2009.05.021 (61) | 68 | 2009 | |
7 | Oerlemans MI, 2012, Basic Res Cardiol, V107, doi: 10.1007/s00395-012-0270-8 (62) | 64 | 2012 | |
8 | Zhang DW, 2009, Science, V325, p332, doi: 10.1126/science.1172308 (63) | 63 | 2009 | |
9 | Degterev A, 2008, Nat Chem Biol, V4, p313, doi: 10.1038/nchembio.83 (64) | 61 | 2008 | |
10 | Wang H, 2014, Mol Cell, V54, p133, doi: 10.1016/j.molcel.2014.03.003 (65) | 57 | 2014 | |
Pyroptosis | 1 | Shi J, 2015, Nature, V526, p660, doi: 10.1038/nature15514 (66) | 130 | 2015 |
2 | Shi J, 2017, Trends Biochem Sci, V42, p245, doi: 10.1016/j.tibs.2016.10.004 (67) | 107 | 2017 | |
3 | Liu X, 2016, Nature, V535, p153, doi: 10.1038/nature18629 (68) | 99 | 2016 | |
4 | Bergsbaken T, 2009, Nat Rev Microbiol, V7, p99, doi: 10.1038/nrmicro2070 (25) | 85 | 2009 | |
5 | Kayagaki N, 2015, Nature, V526, p666, doi: 10.1038/nature15541 (69) | 72 | 2015 | |
6 | Zhaolin Z, 2019, Cell Proliferat, V52, doi: 10.1111/cpr.12563 (16) | 71 | 2019 | |
7 | Duewell P, 2010, Nature, V464, p1357, doi: 10.1038/nature08938 (70) | 69 | 2010 | |
8 | Ding J, 2016, Nature, V535, p111, doi: 10.1038/nature18590 (71) | 60 | 2016 | |
9 | Wu X, 2018, Cell Death Dis, V9, doi: 10.1038/s41419-017-0257-3 (37) | 57 | 2018 | |
10 | Kawaguchi M, 2011, Circulation, V123, p594, doi: 10.1161/circulationaha.110.982777 (72) | 54 | 2011 | |
Ferroptosis | 1 | Dixon SJ, 2012, Cell, V149, p1060, doi: 10.1016/j.cell.2012.03.042 (73) | 390 | 2012 |
2 | Fang X, 2019, Proc Natl Acad Sci U S A, V116, p2672, doi: 10.1073/pnas.1821022116 (26) | 276 | 2019 | |
3 | Stockwell BR, 2017, Cell, V171, p273, doi: 10.1016/j.cell.2017.09.021 (74) | 197 | 2017 | |
4 | Yang WS, 2014, Cell, V156, p317, doi: 10.1016/j.cell.2013.12.010 (75) | 192 | 2014 | |
5 | Friedmann Angeli JP, 2014, Nat Cell Biol, V16, p1180, doi: 10.1038/ncb3064 (76) | 155 | 2014 | |
6 | Gao M, 2015, Mol Cell, V59, p298, doi: 10.1016/j.molcel.2015.06.011 (43) | 148 | 2015 | |
7 | Doll S, 2017, Nat Chem Biol, V13, p91, doi: 10.1038/nchembio.2239 10.1038/nchembio.2239 (77) | 129 | 2017 | |
8 | Xie Y, 2016, Cell Death Differ, V23, p369, doi: 10.1038/cdd.2015.158 (42) | 121 | 2016 | |
9 | Fang X, 2020, Circ Res, V127, p486, doi: 10.1161/circresaha.120.316509 (45) | 113 | 2020 | |
10 | Baba Y, 2018, Am J Physiol-Heart C, V314, ph659, doi: 10.1152/ajpheart.00452.2017 (2) | 106 | 2018 | |
Autophagy | 1 | Nakai A, 2007, Nat Med, V13, p619, doi: 10.1038/nm1574 (78) | 683 | 2007 |
2 | Matsui Y, 2007, Circ Res, V100, p914, doi: 10.1161/01.res.0000261924.76669.36 (79) | 680 | 2007 | |
3 | Zhu H, 2007, J Clin Invest, V117, p1782, doi: 10.1172/jci27523 (80) | 388 | 2007 | |
4 | Levine B, 2008, Cell, V132, p27, doi: 10.1016/j.cell.2007.12.018 (81) | 377 | 2008 | |
5 | Kim J, 2011, Nat Cell Biol, V13, p132, doi: 10.1038/ncb2152 (82) | 373 | 2011 | |
6 | Mizushima N, 2008, Nature, V451, p1069, doi: 10.1038/nature06639 (83) | 287 | 2008 | |
7 | Yan L, 2005, Proc Natl Acad Sci U S A, V102, p13807, doi: 10.1073/pnas.0506843102 (84) | 256 | 2005 | |
8 | Mizushima N, 2011, Cell, V147, p728, doi: 10.1016/j.cell.2011.10.026 (85) | 254 | 2011 | |
9 | Hamacher-Brady A, 2006, J Biol Chem, V281, p29776, doi: 10.1074/jbc.m603783200 (86) | 232 | 2006 | |
10 | Ma X, 2012, Circulation, V125, p3170, doi: 10.1161/circulationaha.111.041814 (52) | 228 | 2012 |
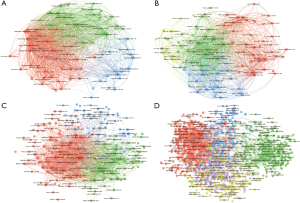
Table S1 and Figure S11 list the top 10 keywords most common keywords for the field of necroptosis in CVD, pyroptosis in CVD, ferroptosis in CVD, and autophagy in CVD. In the field of necroptosis in CVD, the top 10 keywords were “necroptosis”, “apoptosis”, “cell-death”, “oxidative stress”, “necrosis”, “inflammation”, “autophagy”, “heart”, “activation”, and “programmed necrosis”. In the field of pyroptosis CVD, “pyroptosis”, “nlrp3 inflammasome”, “activation”, “apoptosis”, “oxidative stress”, “inflammation”, “cell-death”, “atherosclerosis”, “mechanisms”, and “autophagy” were the top 10 keywords. For ferroptosis in CVD, “ferroptosis”, “oxidative stress”, “cell death”, “iron”, “apoptosis”, “mechanisms”, “lipid peroxidation”, “heart”, “metabolism”, and “autophagy” were the top 10 keywords. Finally, in the field of autophagy in CVD, “autophagy”, “apoptosis”, “oxidative stress”, “heart”, “activation”, “expression”, “heart failure”, “inflammation”, “mechanisms”, and “cell death” were in the top 10 keywords.
Discussion
In our study, a total of 8,146 publications were obtained from the WoSCC. Our results indicated that the number of publications on necroptosis, pyroptosis, ferroptosis, and autophagy in CVD increased annually from 2009 to 2023, especially after 2017. In the field of necroptosis in CVD, the number of publications steadily increased by approximately 10% from 2017 to 2023. Similarly, pyroptosis in CVD experienced rapid growth in publications from 2019 to 2021. In 2021, Shi et al. proposed that the N-terminal of GSDMD is essential for pyroptosis in cardiomyocytes and that the inhibition of GSDMD can attenuate myocardial ischemia-reperfusion injury (MIRI)-induced pyroptosis; this finding, initiated a growth in research related to pyroptosis in CVD (21,87). For ferroptosis in CVD, the number of articles increased annually by 50% between 2017 and 2023. In 2012, ferroptosis, a type of iron-dependent non-apoptotic cell death was discovered in HT-1080 and Calu-1 cells by Dixon et al. (73). In 2019, Fang et al.’s group reported that ferroptosis mediates both chemotherapy and MIRI-induced cardiomyopathy. They have further reported that heme oxygenase 1 breaks down heme and releases free iron, which subsequently causes the production of oxidized lipids in the mitochondrial membrane; moreover, iron chelation therapy can alleviate ferroptosis in cardiomyocytes (26). This article pushed the research on ferroptosis in cardiomyocytes. For autophagy in CVD, the publication output was greater than that of the other types of cell death. The increase in the overall number of publications was particularly high in 2021 and 2022, indicating the high degree of interest in this field.
China ranked first in the number of articles published in this field. In the field of necroptosis in CVD, except for Comenius University Bratislava in Slovakia, the most prolific institutions were all from China, including Harbin Medical University, Zhejiang University, and Fudan University; this indicates that Chinese institutions have shown a growing interest in investigating the role of programmed cell death (PCD) in CVD. As shown in Figures S1-S3, cooperation among countries/regions, as demonstrated via co-authorship, citation, and bibliographic coupling analyses, was observed between China and the United States, with limited cooperation between other nations. As shown in Figures S4-S6, certain institutions were particularly isolated, including Stellenbosch University, the University of Monastir, Kyungpook National University, Ewha Womans University, and the Indian Institute of Technology Kanpur, indicating a lack of collaboration for these organizations. It is strongly recommended that communication between institutions intensifies to facilitate advancements in the fields of autophagy, ferroptosis, necroptosis, and pyroptosis in CVD.
The top 10 most-published authors and co-cited authors in the field of necroptosis in CVD are listed in Table 2 and Figures S2-S5, among whom Adameova A published 22 articles, ranking first in the field of necroptosis in CVD. In the field of pyroptosis in CVD, Ge J and Ye B published each 8 articles, ranking fist in this field, and were followed by Dai S (7 articles). However, the number of citations for Ge J (239 citations) was higher than that for Ye B (211 articles). The leading researcher in the field of autophagy and ferroptosis in CVD was Ren J, with 10 and 120 articles, respectively. In the field of ferroptosis, the number of published papers by Matsui T was less than that of Ren J, but Matsui T was cited more (463 citations) as compared to Ren J (204 citations).
In and co-citation analysis of journals was found that over 50% of the top 10 journals in number of co-citations were the Q1 and Q2 journals (Table 3 and Figure S9, Figure 4). Journal co-citation analysis has also indicated that the top two most-cited journals were Cell and Nature in the fields of ferroptosis in CVD, necroptosis in CVD, and pyroptosis in CVD, respectively, each with over 500 citations. Slightly different from these fields, in the field of autophagy in CVD, the Journal of Biological Chemistry (12,012 citations) was the most frequently cited journal, followed by Circulation Research (11,966 citations) (Table 3 and Figure 5). In summary, the fields of autophagy, ferroptosis, necroptosis, and pyroptosis in CVD are all research hotspots that appear to be growing. Additionally, the cited literature from high-impact journals indicates that these research areas are highly regarded in the academic community.
Based on the data of top 10 co-cited references in the field of necroptosis, pyroptosis, ferroptosis, and autophagy in CVD, the article “CaMKII is a RIP3 substrate mediating ischemia- and oxidative stress-induced myocardial necroptosis” was the most cited article (514 citations) in necroptosis in CVD (24); in pyroptosis in CVD, “Pyroptosis: host cell death and inflammation” was the most cited (2,105 citations) (25); in ferroptosis in CVD, “Ferroptosis: process and function” was the most cited (1,959 citations) (42); and in the field of autophagy in CVD, “Cardioprotection and lifespan extension by the natural polyamine spermidine” was the most cited (681 citations) (27). All the articles related to necroptosis, pyroptosis, and autophagy in CVD were published in Q1 and Q2 journals, with those published on ferroptosis being the exception. The different types of cell death in CVD are at the frontiers of CVD research.
The research hotspots for necroptosis in CVD
Necroptosis, a form of programmed necrosis, was first identified by Degterev et al. in 2005 as a tumor necrosis factor receptor-mediated process that can be inhibited by necrostatin-1 (Nec-1), a small-molecule inhibitor of RIPK1 (60). Once TNF-α binds to tumor necrosis factor receptor I (TNFRI), it will recruit TNFR1-associated death domain protein (TRADD). Subsequently, TRADD recruits RIPK1 through DD-DD interaction. It forms the membrane-bound complex I, which is composed by TRADD, TNFR-associated factor 2, TNFR-associated factor 5, RIP1, and cellular inhibitor of apoptosis 1/2 (22). The conversion of complex I to complex II is dependent on the de-ubiquitination of RIP1 (28). De-ubiquitination of RIP1 promotes the autophosphorylation of RIP1 (Ser161), which subsequently enhances the phosphorylation of RIP3 (Ser227 in humans and Thr231/Ser232 in mice) to activate MLKL protein (Thr357 and Ser358 in humans and Ser358 in mice), forming pores and leading to cell death.
Recent studies have confirmed the detrimental role of necroptosis in MIRI. For instance, RIP3-deficient mice exhibit significantly reduced myocardial inflammation and hypertrophy following MIRI, along with improved cardiac function, including enhanced ejection fraction (24,30). These findings underscore the importance of RIP3 in driving necroptosis-mediated cardiac injury. Supporting this, Zhu et al. reported that RIP3 triggers endoplasmic reticulum (ER) stress, which is associated with elevated intracellular Ca2+ levels [(Ca2+)c] and increased xanthine oxidase (XO) expression. The activation of XO in turn leads to higher levels of cellular reactive oxygen species (ROS), which mediates the opening of the mitochondrial permeability transition pore (mPTP) and induces necroptosis in cardiomyocytes (29). This mechanistic link between RIP3 and mitochondrial dysfunction provides an important avenue for therapeutic intervention.
In addition to the RIP1/RIP3/MLKL pathway, calcium/calmodulin-dependent protein kinase II (CamKII), another RIP3 substrate, triggers opening of the mPTP and myocardial necroptosis, according to a study by Zhang et al. group (24). Furthermore, hesperadin, a CamKII-δ inhibitor, has been found to ameliorate myocardial I/R injury (88). In addition to examining different signaling pathways related to necroptosis, research has focused on the inhibition of necroptosis to improve cardiac protection.
Growing evidence suggests that simultaneous inhibition of necroptosis and apoptosis offers synergistic protection against myocardial I/R injury. It has been suggested that a combination of necroptosis and apoptosis inhibition can enhance cardioprotection against myocardial I/R injury (32). Interestingly, another study found that TNF-α-associated factor 2 can protect cardiomyocytes from both apoptosis and necroptosis in those with heart failure (34), which could represent a novel therapeutic target for addressing pathological remodeling and heart failure. The most promising strategies in the death receptor pathway include inhibiting RIPK1 and RIPK3, with RIPK3 potentially providing greater benefit due to its broader range of downstream targets. Necrostatin-1 was the first inhibitor of necroptosis to be identified (60). However, a study has found that necrostatin-1 is not a selective inhibitor of necroptosis due to possessing multiple targets, including indoleamine 2,3-dioxygenase (IDO) (89). Therefore, several possible targets for inhibiting necroptosis may be available, including necrostatin-1 stable (a selective inhibitor of RIP1), GSK’074 (an inhibitor of RIP1 ad RIP3) (90), and necrosulfonamide (a molecule that inactivates necrosomes) (58). However, due to the insoluble nature of GSK’074 and necrosulfonamide, further in depth research is ongoing.
The research hotspots for pyroptosis in CVD
The term “pyroptosis”, first proposed by Cookson and Brennan, combines the Greek words pyro (meaning fire or fever) and ptosis (meaning falling). It describes a type of inflammatory PCT that was first observed in macrophages. Pyroptosis was initially characterized as relying on caspase-1 and GSDMD. In this process, microorganism- and host-derived “danger” signals trigger the formation of a multiprotein complex known as the inflammasome, which results in the processing and activation of caspase-1 (25,91). Mezzaroma et al. reported that the formation of the inflammasome that includes an apoptosis speck-like protein containing a caspase-recruitment domain (ASC), cryopyrin, and caspase-1, in the mouse heart during acute myocardial infarction (AMI) results in additional loss of the functional myocardium, leading to heart failure. In their study, blocking P2X7 and cryopyrin (using RNA silencing or a pharmacological inhibitors) prevented the inflammasome from forming and decreased infarct size and cardiac enlargement following AMI (36). Moreover, the production of ROS is an upstream mechanism involved in the activation of the NLRP3 inflammasome. Studies have found that nicotine-induced ROS production and oxidative stress are likely upstream mechanisms driving the activation of the NLRP3 inflammasome, a process that can be counteracted by N-acetyl-cysteine (NAC), a ROS inhibitor (16,37,92).
Another target that can initialize pyroptosis is GSDMD. The activated form of GSDMD, comprising an N-terminal domain, has the capability to aggregate and create pores within the cell membrane, which causes the membrane to disrupt, potentially triggering cell death, while also facilitating the release of inflammatory agents such as interleukin (IL)-1β and IL-18. Previous studies have found that the pyroptosis of vascular smooth muscle cells (VSMCs) leads to unstable atherosclerotic plaques and acute coronary syndrome, while the pyroptosis of monocytes and macrophages intensifies inflammation and drives the development of various CVDs (28,38,40,41). However, in 2021, Shi et al. proposed that during myocardial I/R injury, the principal occurrence was cardiomyocyte pyroptosis facilitated by GSDMD and that the inhibition of GSDMD can reduce cardiomyocyte pyroptosis in this process (21,87).
Cleavage of GSDME by caspase-3 can trigger pyroptosis in some cancer cells following chemotherapy, a phenomenon known as noncanonical pyroptosis. In 2020, Zheng et al. reported that doxorubicin (DOX)-induced cardiomyocyte pyroptosis is mediated by GSDME and activated by the upregulation of Bnip3 and cleaved caspase-3 (93). Their findings indicate that targeting pyroptosis could be effective in reducing DOX-induced cardiomyocyte injury.
The research hotspots for ferroptosis in CVD
As a form of regulated cell death, ferroptosis is mediated by the iron-dependent accumulation of lipid hydroperoxides. Iron functions as a cofactor in heme and iron-sulfur cluster-containing proteins that regulate essential processes, such as oxygen transport and oxidative phosphorylation (42). Mice that lack ferritin heavy chain (FTH) specifically in either myocytes or cardiomyocytes exhibit altered cardiac iron homeostasis and develop mild cardiomyopathy at young age. These mice having reduced cardiac iron levels and dietary iron supplementation are affected by severe left ventricular hypertrophy and heart injury due to ferroptosis. This damage can be mitigated either by overexpressing Slc7a11 or administering the ferroptosis inhibitor ferrostatin-1 to the mice (45).
Notably, cystine/glutamate antiporter (System Xc−), GPX4, and glutathione (GSH) (collectively referred to as the system Xc−GSH–GPX4 axis) are crucial to prevent the lipid peroxidation-induced ferroptosis (15). Fang et al.’s group found that iron-dependent ferroptosis was a greater contributor to DOX-induced cardiomyopathy than were other known forms of regulated cell death. Moreover, they found that the activation of Nrf2 leads to the upregulation of Hmox1, resulting in heme degradation in the heart. This process releases free iron, which accumulates in the mitochondria and causes lipid peroxidation (26). A variety of inhibitors and promoters related to ferroptosis have been identified thus far. Liu et al. reported that puerarin, one of the most abundant phytoestrogens with antioxidant and other properties, exerts protective effects against cardiomyocyte hypertrophy through ferroptosis mitigation in rats (48). Transferrin transport and the cellular metabolic process of glutaminolysis are crucial for the ferroptosis induced by the lack of all amino acids or cystine alone. In another study, it was demonstrated that targeting glutaminolysis could be an effective therapeutic strategy for treating heart injury resulting from I/R injury (43). Other research indicates that mammalian target of rapamycin (mTOR) is essential and sufficient to prevent iron-mediated cell death (2). The overexpression of mTOR prevents cell death resulting from excess of iron and ferroptosis, while the absence of mTOR amplifies cell death induced by excessive iron and ferroptosis in cardiomyocytes.
Doll et al. and Bersuker et al. identified that FSP1, in addition to the canonical GSH-based GPX4 pathway, is a crucial part of a non-mitochondrial CoQ antioxidant system that regulates phospholipid peroxidation and ferroptosis (94,95). Meanwhile, Qiu et al.’s group reported that idebenone, a novel ferroptosis inhibitor, stabilizes FSP1 protein levels by inhibiting its ubiquitination degradation, resulting in the attenuation of DOX-induced cardiotoxicity (96). Untargeted metabolomic analysis identified dihydroorotate dehydrogenase-coenzyme Q (DHODH-CoQ) as another crucial anti-ferroptotic pathway (97). Zhu et al. found that the suppression of Cirbp expression, due to aging, weakens the cardioprotective effects of hypothermia by diminishing DHODH-mediated ferroptosis defense, leading to increased ferroptosis in aged donor hearts after transplantation (98).
The research hotspot for autophagy in CVD
Autophagy, derived from the Greek words auto (oneself) and phagy (to eat), describes any cellular degradation pathway that transports cytoplasmic material to the lysosome (57,99). Autophagy is an intracellular process responsible for the bulk degradation of proteins and organelles. The role of autophagy varies significantly across different stages of disease progression and among different cell types (10). In the cardiomyocytes, autophagy is triggered by myocardial ischemia. Autophagy can be divided into different types including chaperone-mediated autophagy, microautophagy, and macroautophagy (49,55). Hamacher-Brady et al. examined the role of macroautophagy in myocardial ischemia reperfusion injury and found that autophagic flux is disrupted at both the initiation and degradation stages; they thus concluded that enhancing autophagy represents a potent and—at that time—previously unrecognized protective mechanism against I/R injury in heart cells (86). Eisenberg et al. reported that spermidine feeding might enhance cardiac autophagy, mitophagy, and prevent cardiac hypertrophy via Atg5 (27). However, a different study found that in different processes of myocardial I/R, the role of autophagy varies. Autophagy may exert a protective effect during ischemia, but it can be detrimental during reperfusion (79). Additionally, Ma et al. reported that re-oxygenation caused more death in neonatal rat cardiomyocytes than did hypoxia alone and significantly increased the number of autophagosomes but not autolysosomes (52). Impaired autophagosome homeostasis can be influenced by ROS, which leads to a decrease of LAMP2 and an increase of BECLIN-1, resulting in a greater degree of cardiomyocyte death.
In addition, several mitochondria-related proteins have been investigated in their relation to autophagy. Recent discoveries have highlighted the significant role of the E3 ubiquitin ligase Parkin in marking damaged mitochondria for removal through the process of autophagy. Kubli et al. found that Parkin-knockout mice exhibited reduced survival and developed larger infarcts compared to wild-type mice following infarction. Notably, in wild-type mice, the expression of Parkin and mitochondrial autophagy (mitophagy) rapidly increased in the border zone of the infarct (53). Dynamin-related protein 1 (Drp1) regulates mitochondrial fission sites. One study found that Drp1 physically interacts with Bcl-2/Bcl-xL and that the downregulation of Drp1 enhances the interaction between Beclin1 and Bcl-2/Bcl-xL, which in turn leads to the suppression of autophagy and exacerbates myocardial injury in response to I/R (50).
Future outlook
While preclinical advances have shed light on the importance of PCD in CVD, overcoming these translational barriers will be crucial to unlocking their therapeutic potential. A major limitation is the lack of specific and safe pharmacological modulators for each PCD pathway. For example, while necrostatins (e.g., necrostatin-1) are widely used to inhibit RIPK1-mediated necroptosis in preclinical studies, their off-target effects and limited pharmacokinetics have hindered clinical development (100). Similarly, although ferroptosis inhibitors like ferrostatin-1 and liproxstatin-1 show promise in animal models, none have advanced to clinical trials in cardiovascular settings (92). Only through further optimization and rigorous testing of these compounds will we be able to conduct meaningful human studies to answer the question of whether the inhibition of ferroptosis, apoptosis, or other forms of cell death in CVD will translate into clinical benefit.
Limitation
Our study is the first study to employ a bibliometric analysis in the fields of necroptosis in CVD, pyroptosis in CVD, ferroptosis in CVD, and autophagy in CVD, yet several limitations should be noted. First, the literature we reviewed was published from January 1, 2009 to December 31, 2023. However, since the WoSCC is continually updated, our search results may differ from the current number of relevant publications. Second, the analysis was solely based on the WoSCC, and relevant studies indexed in other databases or within the grey literature might have been missed, potentially introducing selection bias. Third, as bibliometric analysis is addressed based on keyword extraction, the results might have been affected by the incomplete extraction of keywords. Lastly, although we recognized interdisciplinary collaboration as a key driver of progress in this field, our current analysis did not delve deeply into the nature of existing collaborations across disciplines such as cardiology, molecular biology, and computational sciences—an area that deserves further investigation in future studies. Nonetheless, our analysis could effectively capture the trends, countries/regions, institutions, journals, authors, and co-citations of publications on autophagy, ferroptosis, necroptosis, pyroptosis in the context of CVD.
Conclusions
Our study provides a bibliometric analysis of the literature on autophagy, ferroptosis, necroptosis, and pyroptosis in the context of CVD, examining the authors, institutions, countries/regions, and high-quality publications. Our findings suggest that we should strengthen communication between difference institutions in the future. The bulk of research in this field has focused on the pathways, crosstalk, and inhibitors of the different types of PCD in CVD. Further examination of different cell death processes through clinical trials is warranted.
Acknowledgments
None.
Footnote
Reporting Checklist: The authors have completed the BIBLIO reporting checklist. Available at https://jtd.amegroups.com/article/view/10.21037/jtd-2025-682/rc
Peer Review File: Available at https://jtd.amegroups.com/article/view/10.21037/jtd-2025-682/prf
Funding: This work received financial support from various sources, including
Conflicts of Interest: All authors have completed the ICMJE uniform disclosure form (available at https://jtd.amegroups.com/article/view/10.21037/jtd-2025-682/coif). The authors have no conflicts of interest to declare.
Ethical Statement: The authors are accountable for all aspects of the work in ensuring that questions related to the accuracy or integrity of any part of the work are appropriately investigated and resolved.
Open Access Statement: This is an Open Access article distributed in accordance with the Creative Commons Attribution-NonCommercial-NoDerivs 4.0 International License (CC BY-NC-ND 4.0), which permits the non-commercial replication and distribution of the article with the strict proviso that no changes or edits are made and the original work is properly cited (including links to both the formal publication through the relevant DOI and the license). See: https://creativecommons.org/licenses/by-nc-nd/4.0/.
References
- Yusuf S, Joseph P, Rangarajan S, et al. Modifiable risk factors, cardiovascular disease, and mortality in 155 722 individuals from 21 high-income, middle-income, and low-income countries (PURE): a prospective cohort study. Lancet 2020;395:795-808. [Crossref] [PubMed]
- Baba Y, Higa JK, Shimada BK, et al. Protective effects of the mechanistic target of rapamycin against excess iron and ferroptosis in cardiomyocytes. Am J Physiol Heart Circ Physiol 2018;314:H659-68. [Crossref] [PubMed]
- Christidi E, Brunham LR. Regulated cell death pathways in doxorubicin-induced cardiotoxicity. Cell Death Dis 2021;12:339. [Crossref] [PubMed]
- Ohsumi Y. Historical landmarks of autophagy research. Cell Res 2014;24:9-23. [Crossref] [PubMed]
- Grootjans S, Vanden Berghe T, Vandenabeele P. Initiation and execution mechanisms of necroptosis: an overview. Cell Death Differ 2017;24:1184-95. [Crossref] [PubMed]
- Fang W, Xie S, Deng W. Ferroptosis mechanisms and regulations in cardiovascular diseases in the past, present, and future. Cell Biol Toxicol 2024;40:17. [Crossref] [PubMed]
- Forcina GC, Dixon SJ. GPX4 at the Crossroads of Lipid Homeostasis and Ferroptosis. Proteomics 2019;19:e1800311. [Crossref] [PubMed]
- Xia W, Li Y, Wu M, et al. Gasdermin E deficiency attenuates acute kidney injury by inhibiting pyroptosis and inflammation. Cell Death Dis 2021;12:139. [Crossref] [PubMed]
- Xiang Q, Yi X, Zhu XH, et al. Regulated cell death in myocardial ischemia-reperfusion injury. Trends Endocrinol Metab 2024;35:219-34. [Crossref] [PubMed]
- Adkar SS, Leeper NJ. Efferocytosis in atherosclerosis. Nat Rev Cardiol 2024;21:762-79. [Crossref] [PubMed]
- Santovito D, Steffens S, Barachini S, et al. Autophagy, innate immunity, and cardiac disease. Front Cell Dev Biol 2023;11:1149409. [Crossref] [PubMed]
- Shirakabe A, Ikeda Y, Sciarretta S, et al. Aging and Autophagy in the Heart. Circ Res 2016;118:1563-76. [Crossref] [PubMed]
- Henderson JM, Weber C, Santovito D. Beyond Self-Recycling: Cell-Specific Role of Autophagy in Atherosclerosis. Cells 2021;10:625. [Crossref] [PubMed]
- Gupta K, Phan N, Wang Q, et al. Necroptosis in cardiovascular disease - a new therapeutic target. J Mol Cell Cardiol 2018;118:26-35. [Crossref] [PubMed]
- Fatima S, Zhou H, Chen Y, et al. Role of ferroptosis in the pathogenesis of heart disease. Front Physiol 2024;15:1450656. [Crossref] [PubMed]
- Zhaolin Z, Guohua L, Shiyuan W, et al. Role of pyroptosis in cardiovascular disease. Cell Prolif 2019;52:e12563. [Crossref] [PubMed]
- Szobi A, Rajtik T, Adameova A. Effects of necrostatin-1, an inhibitor of necroptosis, and its inactive analogue Nec-1i on basal cardiovascular function. Physiol Res 2016;65:861-5. [Crossref] [PubMed]
- Erdogmus Ozgen Z, Erdinc M, Kelle İ, et al. Protective effects of necrostatin-1 on doxorubicin-induced cardiotoxicity in rat heart. Hum Exp Toxicol 2022;41:9603271211066066. [Crossref] [PubMed]
- Wang Y, Zhao Y, Ye T, et al. Ferroptosis Signaling and Regulators in Atherosclerosis. Front Cell Dev Biol 2021;9:809457. [Crossref] [PubMed]
- Hatcher HC, Singh RN, Torti FM, et al. Synthetic and natural iron chelators: therapeutic potential and clinical use. Future Med Chem 2009;1:1643-70. [Crossref] [PubMed]
- Shi H, Gao Y, Dong Z, et al. GSDMD-Mediated Cardiomyocyte Pyroptosis Promotes Myocardial I/R Injury. Circ Res 2021;129:383-96. [Crossref] [PubMed]
- Adameova A, Goncalvesova E, Szobi A, et al. Necroptotic cell death in failing heart: relevance and proposed mechanisms. Heart Fail Rev 2016;21:213-21. [Crossref] [PubMed]
- Kokol P, Blažun Vošner H, Završnik J. Application of bibliometrics in medicine: a historical bibliometrics analysis. Health Info Libr J 2021;38:125-38. [Crossref] [PubMed]
- Zhang T, Zhang Y, Cui M, et al. CaMKII is a RIP3 substrate mediating ischemia- and oxidative stress-induced myocardial necroptosis. Nat Med 2016;22:175-82. [Crossref] [PubMed]
- Bergsbaken T, Fink SL, Cookson BT. Pyroptosis: host cell death and inflammation. Nat Rev Microbiol 2009;7:99-109. [Crossref] [PubMed]
- Fang X, Wang H, Han D, et al. Ferroptosis as a target for protection against cardiomyopathy. Proc Natl Acad Sci U S A 2019;116:2672-80. [Crossref] [PubMed]
- Eisenberg T, Abdellatif M, Schroeder S, et al. Cardioprotection and lifespan extension by the natural polyamine spermidine. Nat Med 2016;22:1428-38. [Crossref] [PubMed]
- Del Re DP, Amgalan D, Linkermann A, et al. Fundamental Mechanisms of Regulated Cell Death and Implications for Heart Disease. Physiol Rev 2019;99:1765-817. [Crossref] [PubMed]
- Zhu P, Hu S, Jin Q, et al. Ripk3 promotes ER stress-induced necroptosis in cardiac IR injury: A mechanism involving calcium overload/XO/ROS/mPTP pathway. Redox Biol 2018;16:157-68. [Crossref] [PubMed]
- Luedde M, Lutz M, Carter N, et al. RIP3, a kinase promoting necroptotic cell death, mediates adverse remodelling after myocardial infarction. Cardiovasc Res 2014;103:206-16. [Crossref] [PubMed]
- Qin D, Wang X, Li Y, et al. MicroRNA-223-5p and -3p Cooperatively Suppress Necroptosis in Ischemic/Reperfused Hearts. J Biol Chem 2016;291:20247-59. [Crossref] [PubMed]
- Koshinuma S, Miyamae M, Kaneda K, et al. Combination of necroptosis and apoptosis inhibition enhances cardioprotection against myocardial ischemia-reperfusion injury. J Anesth 2014;28:235-41. [Crossref] [PubMed]
- Zhe-Wei S, Li-Sha G, Yue-Chun L. The Role of Necroptosis in Cardiovascular Disease. Front Pharmacol 2018;9:721. [Crossref] [PubMed]
- Guo X, Yin H, Li L, et al. Cardioprotective Role of Tumor Necrosis Factor Receptor-Associated Factor 2 by Suppressing Apoptosis and Necroptosis. Circulation 2017;136:729-42. [Crossref] [PubMed]
- Szobi A, Gonçalvesová E, Varga ZV, et al. Analysis of necroptotic proteins in failing human hearts. J Transl Med 2017;15:86. [Crossref] [PubMed]
- Mezzaroma E, Toldo S, Farkas D, et al. The inflammasome promotes adverse cardiac remodeling following acute myocardial infarction in the mouse. Proc Natl Acad Sci U S A 2011;108:19725-30. [Crossref] [PubMed]
- Wu X, Zhang H, Qi W, et al. Nicotine promotes atherosclerosis via ROS-NLRP3-mediated endothelial cell pyroptosis. Cell Death Dis 2018;9:171. [Crossref] [PubMed]
- Toldo S, Mauro AG, Cutter Z, et al. Inflammasome, pyroptosis, and cytokines in myocardial ischemia-reperfusion injury. Am J Physiol Heart Circ Physiol 2018;315:H1553-68. [Crossref] [PubMed]
- Li X, Du N, Zhang Q, et al. MicroRNA-30d regulates cardiomyocyte pyroptosis by directly targeting foxo3a in diabetic cardiomyopathy. Cell Death Dis 2014;5:e1479. [Crossref] [PubMed]
- Zeng C, Wang R, Tan H. Role of Pyroptosis in Cardiovascular Diseases and its Therapeutic Implications. Int J Biol Sci 2019;15:1345-57. [Crossref] [PubMed]
- Jia C, Chen H, Zhang J, et al. Role of pyroptosis in cardiovascular diseases. Int Immunopharmacol 2019;67:311-8. [Crossref] [PubMed]
- Xie Y, Hou W, Song X, et al. Ferroptosis: process and function. Cell Death Differ 2016;23:369-79. [Crossref] [PubMed]
- Gao M, Monian P, Quadri N, et al. Glutaminolysis and Transferrin Regulate Ferroptosis. Mol Cell 2015;59:298-308. [Crossref] [PubMed]
- Dodson M, Castro-Portuguez R, Zhang DD. NRF2 plays a critical role in mitigating lipid peroxidation and ferroptosis. Redox Biol 2019;23:101107. [Crossref] [PubMed]
- Fang X, Cai Z, Wang H, et al. Loss of Cardiac Ferritin H Facilitates Cardiomyopathy via Slc7a11-Mediated Ferroptosis. Circ Res 2020;127:486-501. [Crossref] [PubMed]
- Li W, Feng G, Gauthier JM, et al. Ferroptotic cell death and TLR4/Trif signaling initiate neutrophil recruitment after heart transplantation. J Clin Invest 2019;129:2293-304. [Crossref] [PubMed]
- Park TJ, Park JH, Lee GS, et al. Quantitative proteomic analyses reveal that GPX4 downregulation during myocardial infarction contributes to ferroptosis in cardiomyocytes. Cell Death Dis 2019;10:835. [Crossref] [PubMed]
- Liu B, Zhao C, Li H, et al. Puerarin protects against heart failure induced by pressure overload through mitigation of ferroptosis. Biochem Biophys Res Commun 2018;497:233-40. [Crossref] [PubMed]
- Bravo-San Pedro JM, Kroemer G, Galluzzi L. Autophagy and Mitophagy in Cardiovascular Disease. Circ Res 2017;120:1812-24. [Crossref] [PubMed]
- Ikeda Y, Shirakabe A, Maejima Y, et al. Endogenous Drp1 mediates mitochondrial autophagy and protects the heart against energy stress. Circ Res 2015;116:264-78. [Crossref] [PubMed]
- Xie Z, Lau K, Eby B, et al. Improvement of cardiac functions by chronic metformin treatment is associated with enhanced cardiac autophagy in diabetic OVE26 mice. Diabetes 2011;60:1770-8. [Crossref] [PubMed]
- Ma X, Liu H, Foyil SR, et al. Impaired autophagosome clearance contributes to cardiomyocyte death in ischemia/reperfusion injury. Circulation 2012;125:3170-81. [Crossref] [PubMed]
- Kubli DA, Zhang X, Lee Y, et al. Parkin protein deficiency exacerbates cardiac injury and reduces survival following myocardial infarction. J Biol Chem 2013;288:915-26. [Crossref] [PubMed]
- Taneike M, Yamaguchi O, Nakai A, et al. Inhibition of autophagy in the heart induces age-related cardiomyopathy. Autophagy 2010;6:600-6. [Crossref] [PubMed]
- Sciarretta S, Maejima Y, Zablocki D, et al. The Role of Autophagy in the Heart. Annu Rev Physiol 2018;80:1-26. [Crossref] [PubMed]
- Nishida K, Kyoi S, Yamaguchi O, et al. The role of autophagy in the heart. Cell Death Differ 2009;16:31-8. [Crossref] [PubMed]
- Gustafsson AB, Gottlieb RA. Autophagy in ischemic heart disease. Circ Res 2009;104:150-8. [Crossref] [PubMed]
- Sun L, Wang H, Wang Z, et al. Mixed lineage kinase domain-like protein mediates necrosis signaling downstream of RIP3 kinase. Cell 2012;148:213-27. [Crossref] [PubMed]
- Cho YS, Challa S, Moquin D, et al. Phosphorylation-driven assembly of the RIP1-RIP3 complex regulates programmed necrosis and virus-induced inflammation. Cell 2009;137:1112-23. [Crossref] [PubMed]
- Degterev A, Huang Z, Boyce M, et al. Chemical inhibitor of nonapoptotic cell death with therapeutic potential for ischemic brain injury. Nat Chem Biol 2005;1:112-9. [Crossref] [PubMed]
- He S, Wang L, Miao L, et al. Receptor interacting protein kinase-3 determines cellular necrotic response to TNF-α. 2009;137:1100-11.
- Oerlemans MI, Liu J, Arslan F, et al. Inhibition of RIP1-dependent necrosis prevents adverse cardiac remodeling after myocardial ischemia-reperfusion in vivo. Basic Res Cardiol 2012;107:270. [Crossref] [PubMed]
- Zhang DW, Shao J, Lin J, et al. RIP3, an energy metabolism regulator that switches TNF-induced cell death from apoptosis to necrosis. Science 2009;325:332-6. [Crossref] [PubMed]
- Degterev A, Hitomi J, Germscheid M, et al. Identification of RIP1 kinase as a specific cellular target of necrostatins. Nat Chem Biol 2008;4:313-21. [Crossref] [PubMed]
- Wang H, Sun L, Su L, et al. Mixed lineage kinase domain-like protein MLKL causes necrotic membrane disruption upon phosphorylation by RIP3. Mol Cell 2014;54:133-46. [Crossref] [PubMed]
- Shi J, Zhao Y, Wang K, et al. Cleavage of GSDMD by inflammatory caspases determines pyroptotic cell death. Nature 2015;526:660-5. [Crossref] [PubMed]
- Shi J, Gao W, Shao F. Pyroptosis: Gasdermin-Mediated Programmed Necrotic Cell Death. Trends Biochem Sci 2017;42:245-54. [Crossref] [PubMed]
- Liu X, Zhang Z, Ruan J, et al. Inflammasome-activated gasdermin D causes pyroptosis by forming membrane pores. Nature 2016;535:153-8. [Crossref] [PubMed]
- Kayagaki N, Stowe IB, Lee BL, et al. Caspase-11 cleaves gasdermin D for non-canonical inflammasome signalling. Nature 2015;526:666-71. [Crossref] [PubMed]
- Duewell P, Kono H, Rayner KJ, et al. NLRP3 inflammasomes are required for atherogenesis and activated by cholesterol crystals. Nature 2010;464:1357-61. [Crossref] [PubMed]
- Ding J, Wang K, Liu W, et al. Pore-forming activity and structural autoinhibition of the gasdermin family. Nature 2016;535:111-6. [Crossref] [PubMed]
- Kawaguchi M, Takahashi M, Hata T, et al. Inflammasome activation of cardiac fibroblasts is essential for myocardial ischemia/reperfusion injury. Circulation 2011;123:594-604. [Crossref] [PubMed]
- Dixon SJ, Lemberg KM, Lamprecht MR, et al. Ferroptosis: an iron-dependent form of nonapoptotic cell death. Cell 2012;149:1060-72. [Crossref] [PubMed]
- Stockwell BR, Friedmann Angeli JP, Bayir H, et al. Ferroptosis: A Regulated Cell Death Nexus Linking Metabolism, Redox Biology, and Disease. Cell 2017;171:273-85. [Crossref] [PubMed]
- Yang WS. Regulation of ferroptotic cancer cell death by GPX4. Cell 2014;156:317-31. [Crossref] [PubMed]
- Friedmann Angeli JP, Schneider M, Proneth B, et al. Inactivation of the ferroptosis regulator Gpx4 triggers acute renal failure in mice. Nat Cell Biol 2014;16:1180-91. [Crossref] [PubMed]
- Doll S, Proneth B, Tyurina YY, et al. ACSL4 dictates ferroptosis sensitivity by shaping cellular lipid composition. Nat Chem Biol 2017;13:91-8. [Crossref] [PubMed]
- Nakai A, Yamaguchi O, Takeda T, et al. The role of autophagy in cardiomyocytes in the basal state and in response to hemodynamic stress. Nat Med 2007;13:619-24. [Crossref] [PubMed]
- Matsui Y, Takagi H, Qu X, et al. Distinct roles of autophagy in the heart during ischemia and reperfusion: roles of AMP-activated protein kinase and Beclin 1 in mediating autophagy. Circ Res 2007;100:914-22. [Crossref] [PubMed]
- Zhu H, Tannous P, Johnstone JL, et al. Cardiac autophagy is a maladaptive response to hemodynamic stress. J Clin Invest 2007;117:1782-93. [Crossref] [PubMed]
- Levine B, Kroemer G. Autophagy in the pathogenesis of disease. Cell 2008;132:27-42. [Crossref] [PubMed]
- Kim J, Kundu M, Viollet B, et al. AMPK and mTOR regulate autophagy through direct phosphorylation of Ulk1. Nat Cell Biol 2011;13:132-41. [Crossref] [PubMed]
- Mizushima N, Levine B, Cuervo AM, et al. Autophagy fights disease through cellular self-digestion. Nature 2008;451:1069-75. [Crossref] [PubMed]
- Yan L, Vatner DE, Kim SJ, et al. Autophagy in chronically ischemic myocardium. Proc Natl Acad Sci U S A 2005;102:13807-12. [Crossref] [PubMed]
- Mizushima N, Komatsu M. Autophagy: renovation of cells and tissues. Cell 2011;147:728-41. [Crossref] [PubMed]
- Hamacher-Brady A, Brady NR, Gottlieb RA. Enhancing macroautophagy protects against ischemia/reperfusion injury in cardiac myocytes. J Biol Chem 2006;281:29776-87. [Crossref] [PubMed]
- Gao Y, Shi H, Dong Z, et al. Current knowledge of pyroptosis in heart diseases. J Mol Cell Cardiol 2022;171:81-9. [Crossref] [PubMed]
- Zhang J, Liang R, Wang K, et al. Novel CaMKII-δ Inhibitor Hesperadin Exerts Dual Functions to Ameliorate Cardiac Ischemia/Reperfusion Injury and Inhibit Tumor Growth. Circulation 2022;145:1154-68. [Crossref] [PubMed]
- Takahashi N, Duprez L, Grootjans S, et al. Necrostatin-1 analogues: critical issues on the specificity, activity and in vivo use in experimental disease models. Cell Death Dis 2012;3:e437. [Crossref] [PubMed]
- Zhou T, Wang Q, Phan N, et al. Identification of a novel class of RIP1/RIP3 dual inhibitors that impede cell death and inflammation in mouse abdominal aortic aneurysm models. Cell Death Dis 2019;10:226. [Crossref] [PubMed]
- Dai F, Li X, Li X, et al. Caspase-1 Abrogates the Salutary Effects of Hypertrophic Preconditioning in Pressure Overload Hearts via IL-1β and IL-18. Front Mol Biosci 2021;8:641585. [Crossref] [PubMed]
- Ryabov VV, Maslov LN, Vyshlov EV, et al. Ferroptosis, a Regulated Form of Cell Death, as a Target for the Development of Novel Drugs Preventing Ischemia/Reperfusion of Cardiac Injury, Cardiomyopathy and Stress-Induced Cardiac Injury. Int J Mol Sci 2024;25:897. [Crossref] [PubMed]
- Zheng X, Zhong T, Ma Y, et al. Bnip3 mediates doxorubicin-induced cardiomyocyte pyroptosis via caspase-3/GSDME. Life Sci 2020;242:117186. [Crossref] [PubMed]
- Doll S, Freitas FP, Shah R, et al. FSP1 is a glutathione-independent ferroptosis suppressor. Nature 2019;575:693-8. [Crossref] [PubMed]
- Bersuker K, Hendricks JM, Li Z, et al. The CoQ oxidoreductase FSP1 acts parallel to GPX4 to inhibit ferroptosis. Nature 2019;575:688-92. [Crossref] [PubMed]
- Qiu H, Huang S, Liu Y, et al. Idebenone alleviates doxorubicin-induced cardiotoxicity by stabilizing FSP1 to inhibit ferroptosis. Acta Pharm Sin B 2024;14:2581-97. [Crossref] [PubMed]
- Mao C, Liu X, Zhang Y, et al. DHODH-mediated ferroptosis defence is a targetable vulnerability in cancer. Nature 2021;593:586-90. [Crossref] [PubMed]
- Zhu Y, Jiang C, He J, et al. Cirbp suppression compromises DHODH-mediated ferroptosis defense and attenuates hypothermic cardioprotection in an aged donor transplantation model. J Clin Invest 2024;134:e175645. [Crossref] [PubMed]
- Tang Y, Xu W, Liu Y, et al. Autophagy protects mitochondrial health in heart failure. Heart Fail Rev 2024;29:113-23. [Crossref] [PubMed]
- Yao K, Shi Z, Zhao F, et al. RIPK1 in necroptosis and recent progress in related pharmaceutics. Front Immunol 2025;16:1480027. [Crossref] [PubMed]
(English Language Editor: J. Gray)