The effects of different prone ventilation strategies on mechanical power and respiratory mechanics in acute respiratory distress syndrome patients: a prospective, single-center observational study
Highlight box
Key findings
• The early adoption of lateral-prone ventilation may help mitigate the risk of ventilator-induced lung injury (VILI), and reduced mechanical power (MP) in acute respiratory distress syndrome (ARDS) patients.
What is known, and what is new?
• Mechanical ventilation for ARDS, while essential, risks VILI via stress/strain mechanisms. Prone ventilation is established to reduce VILI by improving oxygenation, alveolar recruitment, and inflammatory mediator reduction, lowering mortality in ARDS and coronavirus disease 2019 patients.
• This study introduces a novel comparison between prone and lateral-prone ventilation strategies. While baseline patient characteristics, Acute Physiology and Chronic Health Evaluation II scores, oxygen saturation to fraction of inspired oxygen, mean arterial pressure, and airway resistance showed no differences, MP—a key VILI risk indicator—significantly differed between groups. This highlights lateral-prone positioning’s distinct mechanical impact, suggesting its potential role in modulating VILI risk.
What is the implication, and what should change now?
• Lateral-prone ventilation should be prioritized in ARDS protocols when MP reduction is critical, particularly in early disease stages. Ventilation strategy algorithms should incorporate MP thresholds alongside oxygenation targets. Real-time MP monitoring should be integrated into critical care ventilators to guide dynamic positioning decisions.
Introduction
Acute respiratory distress syndrome (ARDS) is a severe form of acute lung injury caused by various factors, including pulmonary conditions (e.g., pneumonia and inhalation injury) and extrapulmonary conditions (e.g., sepsis, pancreatitis, and trauma). Global epidemiological study reports that ARDS accounts for 10.4% of total intensive care unit (ICU) admissions, and 23.4% of patients require mechanical ventilation (1). The in-hospital mortality rates for mild, moderate, and severe ARDS are 34.9%, 40.3%, and 46.1%, respectively (2). During the coronavirus disease 2019 (COVID-19) pandemic, the incidence of ARDS surged, with mortality rates for COVID-19-associated ARDS reaching 62%, placing significant strain on healthcare systems and providers worldwide (2).
Mechanical ventilation is critical for maintaining oxygenation and ventilation in ARDS patients. However, high airway pressures and large tidal volumes during mechanical ventilation can cause alveolar overdistension and repetitive collapse, leading to mechanical stress and strain (3). This can trigger ventilator-induced lung injury (VILI), a condition driven by mechanisms such as volutrauma, barotrauma, atelectrauma, and biotrauma (4). The mechanical stress and strain associated with VILI exacerbate lung injury by releasing inflammatory mediators, contributing to systemic inflammatory response syndrome (SIRS), which can progress to multiple organ dysfunction syndrome (MODS) (3,4).
Prone ventilation improves oxygenation and ventilation in ARDS by repositioning patients to reduce alveolar overdistension and promote the recruitment of collapsed alveoli. It enhances ventilation-perfusion matching in gravity-dependent lung regions, improves gas exchange, and reduces the expression of inflammatory mediators. These effects mitigate the risk of VILI and significantly reduce ARDS mortality rates (3-5). Study has shown that prone ventilation improves oxygenation and may reduce mortality in mechanically ventilated COVID-19 ARDS patients (6). Prone ventilation is now a well-established and effective treatment for ARDS (7). However, studies on lateral-prone ventilation are limited, as are comparing prone and lateral-prone strategies regarding VILI risk.
The purpose of this study was to compare the differences of mechanical power (MP) and respiratory parameters related to VILI in ARDS patients under prone ventilation and lateral-prone ventilation, so as to provide data basis for finding more effective ventilation strategies and reducing the risk of VILI in ARDS patients. We present this article in accordance with the TREND reporting checklist (available at https://jtd.amegroups.com/article/view/10.21037/jtd-2025-267/rc).
Methods
Study participants
One hundred and twenty-two patients with moderate-to-severe ARDS admitted to the Department of Critical Care Medicine at Lishui Central Hospital between December 2021 and April 2024 were enrolled in this study. To be eligible for inclusion in this study, the patients had to meet the following inclusion criteria: (I) be aged 18 years or older with a mechanical ventilation duration >48 hours; (II) have been diagnosed with ARDS according to the Berlin Definition 2012, and have an arterial oxygen partial pressure to fractional inspired oxygen (PaO2/FiO2) ratio ≤200 mmHg; and (III) provide written informed consent, which was obtained from either the patients or their legal guardians.
Patients were excluded from the study if they met any of the following exclusion criteria: (I) were pregnant or breastfeeding; (II) had contraindications to prone ventilation, including intracranial hypertension, recent maxillofacial or thoracic surgery, massive hemoptysis, or spinal/pelvic instability; (III) had hemodynamic instability requiring high-dose vasopressors; (IV) had severe pneumothorax or pleural effusion requiring drainage; (V) had a life expectancy of <24 hours, or an active pulmonary or airway hemorrhage; (VI) had severe cardiovascular diseases, such as advanced coronary artery disease, or recent myocardial infarction; (VII) had undergone major surgery in the previous 6 months; (VIII) had severe hepatic or renal dysfunction or failure; and/or (IX) had any other conditions deemed likely to interfere with the study.
This study was conducted in accordance with the Declaration of Helsinki and its subsequent amendments. This prospective study was approved by the Ethics Committee of Lishui Central Hospital (ethics approval No. 2021-311) and informed consent was taken from the patients or their legal guardians.
Interventions
Patients with moderate-to-severe ARDS included in the study were randomly assigned to Group A or Group B via blinded envelope. The following intervention sequences were employed: Group A: sequential ventilation in the supine position, prone position, and lateral-prone position; and Group B: sequential ventilation in the supine position, lateral-prone position, and prone position. The duration of prone ventilation was at least 16 hours per day. All patients were uniformly set to volume-controlled ventilation mode with decelerating flow waveform. Tidal volume was maintained at 6 mL/kg (predicted body weight) for regulation. Positive end-expiratory pressure (PEEP) and fraction of inspired oxygen (FiO2) were adjusted according to the ARDSnet protocol. Ventilator parameters were only modified when driving pressure (DP) exceeded 15 cmH2O or arterial blood gas analysis revealed pH <7.25. Appropriate sedation was administered under adequate analgesia, maintaining a Richmond Agitation Sedation Scale (RASS) score between −3 and −4. High-flow nasal cannula (HFNC) oxygen therapy was consistently applied post-extubation.
Positioning protocols
The following two positioning protocols were employed: (I) the prone positioning protocol: cylindrical cushions were placed under the chest and iliac crests to prevent compression of the thoracoabdominal region; and (II) the lateral-prone positioning protocol: soft cushions were placed under one side of the chest, hips, and knees, and the body position angle was maintained between 15° and 30° to ensure alignment between the torso and head. To minimize the risk of pressure injuries, patients in the lateral-prone position were alternated between the left and right sides every 2 hours.
The schematic timeline of the study workflow is shown in Figure 1, which illustrates the timeline of positional changes corresponding to the same time points for different groups. Each bar represents the ventilation positions at specific time intervals; in Group A, the sequential changes in positions included supine, prone, and lateral-prone ventilation; while in Group B, the sequential changes alternated between supine, lateral-prone, and prone ventilation. The horizontal axis represents the timeline in hours, while the labels indicate the specific positions adopted by each group at corresponding time points.
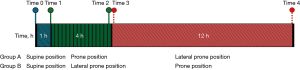
Data measurements
Respiratory mechanics and hemodynamic parameters were measured at predefined time points to assess the effects of different ventilation strategies on ARDS patients. For Group A, the measurements were performed in the supine position, at 1 and 4 hours after transitioning to the prone position, and at the beginning and end of the lateral-prone position. For Group B, the measurements were performed in the supine position, at 1 and 4 hours after transitioning to the lateral-prone position, and at the beginning and end of the prone position.
The measured parameters included: MP, which was calculated using the tidal volume, airway pressure, respiratory rate, inspiratory flow rate, inspiratory-to-expiratory ratio, and PEEP; DP, which was defined as the difference between the plateau pressure and PEEP; static lung compliance (Cstat), which was defined as the tidal volume divided by the DP; airway resistance (Raw), which was defined as the difference between the peak and plateau pressures divided by the inspiratory flow rate; the oxygenation index, which was defined as the oxygen saturation to FiO2 (SpO2/FiO2) ratio; and mean arterial pressure (MAP) and heart rate (HR), which were both continuously monitored.
All measurements were conducted using calibrated equipment (ventilator: Nellcor Puritan Bennett PB840; monitor: PHILIPS MP40) by trained personnel. The devices were calibrated before each use, and the data were recorded directly into an electronic database to ensure accuracy and traceability.
Statistical analysis
All the data were analyzed using SPSS software version 26.0. Data preprocessing included handling missing values, detecting outliers, and data cleaning. The Shapiro-Wilk test was used to assess the normality of the data. For the normally distributed variables, paired t-tests were applied for within-group comparisons, while for the non-normally distributed variables, the Wilcoxon signed-rank test was used. The effects of different ventilation strategies on respiratory and hemodynamic parameters were analyzed by multivariate analysis of variance to evaluate interactions among multiple variables. To assess the associations between MP, Cstat, and the other parameters. Correlation analyses were performed using Pearson’s correlation coefficient for the normally distributed data and Spearman’s rank correlation coefficient for the non-normally distributed data. The results are expressed as the mean ± standard deviation, and a significance level of P<0.05 was considered statistically significant.
Results
Comparison of clinical characteristics
One hundred twenty-two patients diagnosed with moderate-to-severe ARDS (PaO2/FiO2 ≤200 mmHg) were enrolled in this study; Group A comprised 62 patients and Group B comprised 60 patients. There were no statistically significant differences between the two groups in terms of the baseline clinical characteristics, including gender distribution, age, body mass index, Acute Physiology and Chronic Health Evaluation II (APACHE-II) score, and causes of infection (P>0.05).
Given that APACHE-II scores are critical for assessing the severity of illness, any potential influence of these scores was controlled as a confounding factor in the subsequent analyses to ensure the scientific rigor and reliability of the evaluation of the different ventilation strategies. Detailed results are presented in Table 1.
Table 1
Parameters | Group A (n=62) | Group B (n=60) | P value |
---|---|---|---|
Gender | 0.17 | ||
Male | 44 (70.97) | 49 (81.67) | |
Female | 18 (29.03) | 11 (18.33) | |
Age (years) | 76.13±10.51 | 77.72±7.10 | 0.34 |
BMI (kg/m2) | 24.12±3.98 | 25.26±3.96 | 0.09 |
APACHE-II (score) | 23.76±5.90 | 23.65±4.33 | 0.91 |
Type of infection | 0.96 | ||
Bacterial infection | 4 (6.45) | 5 (8.33) | |
Viral infection | 58 (93.55) | 55 (91.67) | |
Hypertension | 14 (22.58) | 19 (31.67) | – |
Hyperlipemia | 22 (35.48) | 15 (25.00) | – |
Diabetes mellitus | 12 (19.35) | 9 (15.00) | – |
COPD | 4 (6.45) | 7 (11.67) | – |
Chronic nephrosis | 6 (9.68) | 9 (15.00) | – |
Others | 4 (6.45) | 1 (1.67) | – |
Data are presented as n (%) or mean ± standard deviation. Group A: sequential ventilation in the supine position, prone position, and lateral-prone position; Group B: sequential ventilation in the supine position, lateral-prone position, and prone position. APACHE-II, Acute Physiology and Chronic Health Evaluation II; BMI, body mass index; COPD, chronic obstructive pulmonary disease.
Effects of positional changes on hemodynamic parameters
This study assessed the effect of different ventilation strategies on hemodynamic parameters, including MAP and HR, in patients with ARDS. No significant differences in MAP variations at any time point were found between the two groups (P>0.05). Table 2 shows the hemodynamic variables in the prone and prone-lateral groups. There were no significant differences in MAP and HR in the two groups. These findings suggest that regardless of the ventilation strategy used, MAP remained relatively stable, supporting the safety and feasibility of positional adjustments in the management of ARDS.
Table 2
MAP | Group A (n=62) | Group B (n=60) | P value |
---|---|---|---|
MAP1 | 82.58±10.21 | 80.37±6.72 | 0.16 |
MAP2 | 81.86±9.35 | 79.92±6.68 | 0.19 |
MAP3 | 82.29±7.44 | 80.18±6.37 | 0.09 |
MAP4 | 82.52±8.42 | 79.88±6.66 | 0.06 |
MAP5 | 81.21±9.33 | 79.15±10.61 | 0.26 |
Data are presented as mean ± standard deviation. Group A: sequential ventilation in the supine position, prone position, and lateral-prone position; Group B: sequential ventilation in the supine position, lateral-prone position, and prone position. MAP, mean arterial pressure.
In relation to HR, no significant differences in HR variations at any time point were found between the two groups (P>0.05) (Table 3). In summary, the lateral-prone ventilation strategy caused minor short-term fluctuations in HR but had no significant effect on the long-term hemodynamic stability, further supporting its safety and clinical applicability.
Table 3
HR | Group A (n=62) | Group B (n=60) | P value |
---|---|---|---|
HR1 | 80.73±19.46 | 79.87±21.49 | 0.82 |
HR2 | 79.47±15.98 | 81.08±21.94 | 0.64 |
HR3 | 80.11±17.84 | 80.70±21.51 | 0.87 |
HR4 | 80.27±17.81 | 80.00±20.44 | 0.94 |
HR5 | 79.73±18.46 | 79.37±19.82 | 0.92 |
Data are presented as mean ± standard deviation. Group A: sequential ventilation in the supine position, prone position, and lateral-prone position; Group B: sequential ventilation in the supine position, lateral-prone position, and prone position. HR, heart rate.
Effects of positional changes on respiratory mechanics
The oxygenation index is a critical parameter reflecting a patient’s oxygenation status and is calculated as the SpO2/FiO2 ratio. A higher oxygenation index value indicates better oxygenation, while a lower value suggests impaired oxygenation. This study evaluated the effects of different ventilation strategies on the oxygenation status of ARDS patients.
The results (Figure 2 and Table 4) showed no significant differences in the SpO2/FiO2 ratio between the two groups following prone or lateral-prone positioning (P>0.05). This finding may be attributed to impaired pulmonary circulation and uneven blood flow distribution in ARDS patients. In the context of systemic hemodynamic instability or cardiac dysfunction, improvements in overall oxygenation may be limited.
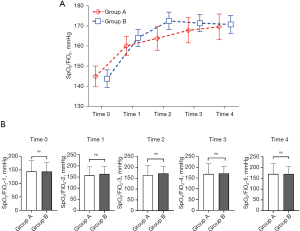
Table 4
Oxygenation index | Group A (n=62) | Group B (n=60) | P value |
---|---|---|---|
SpO2/FiO2-1 | 144.48±39.66 | 143.90±34.11 | 0.93 |
SpO2/FiO2-2 | 158.60±37.42 | 164.13±32.82 | 0.39 |
SpO2/FiO2-3 | 164.80±45.19 | 172.53±33.40 | 0.28 |
SpO2/FiO2-4 | 168.60±47.54 | 171.40±32.70 | 0.71 |
SpO2/FiO2-5 | 170.30±48.50 | 170.75±33.93 | 0.95 |
Data are presented as mean ± standard deviation. Group A: sequential ventilation in the supine position, prone position, and lateral-prone position; Group B: sequential ventilation in the supine position, lateral-prone position, and prone position. SpO2/FiO2, oxygen saturation to fraction of inspired oxygen ratio.
The results (Table 5) indicated that positional changes had no significant effect on Raw in either group (P>0.05), which suggests that positional adjustments exert minimal influence on Raw.
Table 5
Raw | Group A (n=62) | Group B (n=60) | P value |
---|---|---|---|
Raw-1 | 12.59±2.43 | 11.68±3.09 | 0.08 |
Raw-2 | 13.68±2.48 | 12.97±2.75 | 0.14 |
Raw-3 | 13.81±2.26 | 13.07±2.69 | 0.10 |
Raw-4 | 12.34±2.31 | 13.03±2.52 | 0.12 |
Raw-5 | 12.17±2.24 | 12.83±2.47 | 0.12 |
Data are presented as mean ± standard deviation. Group A: sequential ventilation in the supine position, prone position, and lateral-prone position; Group B: sequential ventilation in the supine position, lateral-prone position, and prone position. Raw, airway resistance.
Damage to the alveolar-capillary membrane in ARDS patients significantly reduces lung compliance. Cstat, an indicator of lung elasticity and distensibility, is influenced by airway pressure and lung volume. The results (Table 6) showed that Cstat increased from the baseline across different positions in both groups, particularly after the lateral-prone position, but these changes did not reach the level of statistical significance (P>0.05).
Table 6
Cstat | Group A (n=62) | Group B (n=60) | P value |
---|---|---|---|
Cstat-1 | 34.70±7.36 | 36.20±7.97 | 0.28 |
Cstat-2 | 35.56±6.63 | 38.90±7.75 | 0.08 |
Cstat-3 | 35.88±6.17 | 38.28±7.72 | 0.06 |
Cstat-4 | 35.19±6.87 | 36.33±6.43 | 0.36 |
Cstat-5 | 35.31±7.02 | 37.40±6.63 | 0.09 |
Data are presented as mean ± standard deviation. Group A: sequential ventilation in the supine position, prone position, and lateral-prone position; Group B: sequential ventilation in the supine position, lateral-prone position, and prone position. Cstat, static lung compliance.
The effect of body position changes on pulmonary mechanics
This study evaluated the effects of different ventilatory strategies on pulmonary MP and DP in patients with ARDS. MP reflects the total mechanical load imposed on the lungs during mechanical ventilation, and excessive MP is closely associated with the development of VILI. DP, defined as the pressure difference driving gas into the alveoli during each respiratory cycle, is a key parameter for assessing lung elastic load and the effectiveness of mechanical ventilation.
The results (Figure 3 and Table 7) showed that the lateral-prone position significantly reduced pulmonary MP. Specifically, in Group A, transitioning from the flat-prone position to the lateral-prone position resulted in a significant decrease in MP. Conversely, in Group B, transitioning from the lateral-prone position to the flat-prone position led to a statistically significant increase in MP (P<0.05). Notably, the reduction in MP was more pronounced at 1 and 4 hours after adopting the lateral-prone position (P<0.05). These findings indicate that the lateral-prone position helps alleviate the pulmonary mechanical load by improving ventilation-perfusion matching, increasing alveolar recruitment, and reducing localized alveolar overdistension. Consequently, the mechanical work exerted by the ventilator on the lungs is decreased. These results highlight the potential benefits of the lateral-prone position in reducing the risk of VILI.
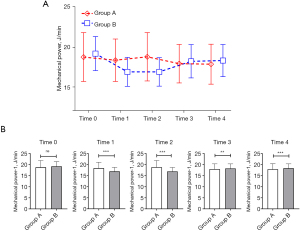
Table 7
MP | Group A (n=62) | Group B (n=60) | P value |
---|---|---|---|
MP-1 | 18.98±3.08 | 19.20±2.13 | 0.66 |
MP-2 | 18.37±2.66 | 16.91±1.81 | <0.001 |
MP-3 | 18.80±3.03 | 16.91±1.78 | <0.001 |
MP-4 | 17.12±2.49 | 18.24±2.08 | 0.008 |
MP-5 | 17.07±1.71 | 18.33±2.01 | <0.001 |
Data are presented as mean ± standard deviation. Group A: sequential ventilation in the supine position, prone position, and lateral-prone position; Group B: sequential ventilation in the supine position, lateral-prone position, and prone position. MP, mechanical power.
The study results (Table 8) indicated that positional changes significantly affected DP in both groups (P<0.05). Prone positioning improved ventilation-perfusion matching, particularly in the dependent lung regions, which might enhance alveolar gas exchange efficiency and reduce the mechanical energy required to maintain the same tidal volume. Lateral-prone positioning may further optimize alveolar expansion and gas distribution, increasing lung compliance and thereby reducing the DP needed for mechanical ventilation. These findings suggest that positional adjustments significantly improve the lung elastic load. The lateral-prone position may alter gas flow patterns in the lungs, reducing air retention and backflow in the airways, thereby enhancing ventilation efficiency and decreasing the MP demand.
Table 8
DP | Group A (n=62) | Group B (n=60) | P value |
---|---|---|---|
DP-1 | 16.42±3.01 | 16.58±2.33 | 0.74 |
DP-2 | 16.11±2.90 | 14.73±2.50 | 0.006 |
DP-3 | 16.13±2.77 | 14.80±2.37 | 0.005 |
DP-4 | 15.60±2.50 | 16.70±1.91 | 0.007 |
DP-5 | 15.52±2.87 | 16.92±2.08 | 0.003 |
Data are presented as mean ± standard deviation. Group A: sequential ventilation in the supine position, prone position, and lateral-prone position; Group B: sequential ventilation in the supine position, lateral-prone position, and prone position. DP, driving pressure.
Comparative outcomes of MP across positional strategies in ARDS patients
This study comprehensively evaluated the clinical outcomes of different ventilation strategies in ARDS patients, including the survival curves and mortality rates. The results (Table 9) indicated that no significant difference in the mortality rates was observed between the two groups (P>0.05).
Table 9
Parameters | Group A (n=62) | Group B (n=60) | P value |
---|---|---|---|
Mortality | 11 (17.74) | 16 (26.67) | 0.24 |
Data are presented as n (%). Group A: sequential ventilation in the supine position, prone position, and lateral-prone position; Group B: sequential ventilation in the supine position, lateral-prone position, and prone position. ARDS, acute respiratory distress syndrome.
However,the log-rank test (Figure 4) revealed that the lateral-prone position offers advantages in improving clinical outcomes for ARDS patients, particularly in terms of prolonging survival. The lateral-prone position reduced MP and the risk of VILI, contributing to improved survival rates to some extent.
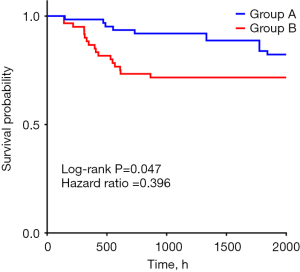
Discussion
ARDS is a form of respiratory failure caused by various factors. It is primarily characterized by alveolar and interstitial edema and heterogeneous regional pathological changes. ARDS commonly occurs in critically ill patients, particularly in the presence of infection, trauma, or severe systemic inflammation (8,9). Mechanical ventilation is a critical intervention for ARDS management that facilitates alveolar recruitment, reduces pulmonary capillary pressure, and improves gas exchange. However, it can also induce VILI, which may exacerbate SIRS, which can progress to MODS (10). Reducing MP in ARDS patients to mitigate ventilator-associated lung injury is thus a major challenge in ARDS management. Lowering the mechanical load exerted on the lungs during mechanical ventilation not only prevents further lung damage but also has the potential to improve the overall outcomes by minimizing systemic inflammatory consequences and preserving organ function.
This study was the first to systematically compare the lung-protective effects of prone and lateral-prone ventilation strategies in ARDS patients. The rationale for selecting the lateral-prone position is its ability to leverage gravity to promote alveolar recruitment, reduce dead space, improve ventilation/perfusion ratios, and decrease cardiac compression on the lungs. These factors collectively optimize ventilation and perfusion matching, enhancing oxygenation efficiency. Alveoli in gravity-dependent regions are more effectively recruited through positional changes, particularly the gravitational effects of the lateral-prone position. This improves gas exchange and reduces the proportion of dead space. The oxygenation index reflects multiple factors and may not fully represent a patient’s clinical status or prognosis. While prone ventilation is widely employed in ARDS management to improve oxygenation, factors such as pulmonary pathology, physiological conditions, and mechanical ventilation settings can sometimes lead to minimal changes in the SpO2/FiO2 ratio. In clinical practice, individualized assessment and tailored treatment strategies are crucial for optimizing oxygenation outcomes in ARDS patients. However, the results of this study revealed no significant difference in the oxygenation index between the lateral-prone and prone positions. This finding could be due to individual variability among the patients, the severity of the disease, and differences in ventilation parameter settings. On the other hand, it may be attributed to the heterogeneous nature of pulmonary lesions in ARDS patients, where the spatial distribution of lung injuries varies significantly across individuals. Furthermore, the lateral-prone positioning protocol lacked individualized adjustments in orientation and angle based on the specific anatomical locations of pulmonary pathologies.
Prone ventilation has also improved oxygenation in patients with acute respiratory failure caused by COVID-19 (11,12). The pathological features of COVID-19, which is characterized by severe alveolar damage, inflammation, and ventilation/perfusion mismatch, leading to hypoxemia, are similar to those of ARDS. By recruiting collapsed alveoli, reducing atelectasis, and optimizing ventilation/perfusion matching, prone ventilation enhances oxygenation levels. Clinical studies have shown that prone positioning significantly improves oxygenation in COVID-19 patients, reduces respiratory rates, and decreases the need for mechanical ventilation (13-15). Similarly, the present study found that lateral-prone ventilation produced even more pronounced effects in COVID-19-associated ARDS, further supporting its potential application in managing acute respiratory failure.
In terms of hemodynamics, no significant differences were observed between the prone and lateral-prone positions in terms of HR, MAP, Cstat, or Raw. The relatively minor effect of prone positioning on intrathoracic pressure and cardiac function can be attributed to the thoracic support provided in this position, which helps distribute intrathoracic pressure evenly, reducing localized pressure concentrations and minimizing hemodynamic effects. In this study, the transient elevation in HR might have been due to the initial adaptive response to the new position, likely mediated by sympathetic nervous system activation. This thoracic support mechanism effectively reduces the mechanical load on the heart, particularly in gravity-dependent regions, thereby maintaining hemodynamic stability. Additionally, the compliance of damaged lung tissue primarily depends on the extent of lung injury, which positional changes are less likely to influence. As a result, no significant differences in compliance were observed between the prone and lateral-prone positions. ARDS patients often exhibit elevated baseline Raw due to pulmonary edema, alveolar destruction, and other pathological alterations. These conditions may offset the potential benefits of positional changes on Raw improvement. Further, positional changes have a relatively limited effect on the patency of large airways, which might explain the absence of significant changes in Raw. These findings suggest that the primary benefit of positional adjustments lies in enhancing oxygenation rather than directly affecting Raw. In clinical practice, ARDS patients with high baseline Raw may require additional therapeutic interventions combined with positional ventilation to achieve more pronounced improvements in respiratory mechanics.
The reduction in Cstat observed in the ARDS patients can be attributed to factors such as alveolar-capillary membrane damage, inflammation, and fibrosis. Positional adjustments may enhance local alveolar ventilation; however, the extent of improvement in compliance appears limited in severe ARDS due to extensive pathological changes throughout the lungs. While the lateral-prone position may improve alveolar recruitment in certain regions, the overall pathological condition of the lungs remains largely unchanged, which might explain the limited effect of the position on Cstat. These findings suggest that positional strategies in ARDS management should primarily aim to improve oxygenation, and their ability to improve lung compliance may be limited. Combining positional adjustments with additional therapeutic interventions may be necessary to achieve better lung function outcomes.
MP reflects the energy expenditure of mechanical ventilation and is a critical parameter for assessing the invasiveness of ventilation and the risk of VILI (14). MP integrates factors such as the tidal volume, respiratory rate, airway pressure, and lung compliance to quantify the physical stress exerted on the lungs during mechanical ventilation. It aids clinicians in optimizing ventilation settings to minimize lung injury. Higher MP is strongly associated with an increased risk of VILI; thus, it is essential to maintain MP in a reasonable range during ARDS treatment (16-19).
DP represents the difference between airway pressure and alveolar pressure, and is often used to measure airway pressure variations during ventilation. At a constant tidal volume, an increase in DP indicates that more energy is being applied to the lungs, heightening the risk of lung injury. Increases in DP are typically accompanied by increases in MP, further escalating the potential for lung damage.
This study showed that the lateral-prone position significantly reduced both MP and DP in ARDS patients. These reductions help mitigate alveolar overdistension and repetitive collapse, improving overall lung ventilation and decreasing the risk of VILI. These findings underscore the role of the lateral-prone position in optimizing mechanical ventilation and protecting lung integrity in ARDS patients.
This study showed the beneficial effects of lateral-prone ventilation on MP; however, it had several limitations that should be noted. For example, this study did not evaluate the impact of the two positioning strategies on critical clinical outcomes, including mortality rate, tracheal reintubation rate, duration of mechanical ventilation, and ICU length of stay. Future investigations hope to validate the effects of these positions on patient-centered outcomes through multicenter, large-sample randomized controlled trials (RCTs). Furthermore, the current assessment of respiratory mechanics was limited to parameters such as SpO2/FiO2 ratio, Raw, Cstat, and DP. Subsequent research aims to incorporate advanced visualization modalities—including electrical impedance tomography (EIT), lung ultrasonography, and bedside computed tomography (CT) scans—to comprehensively evaluate positional effects on respiratory mechanics, thereby guiding personalized prone positioning strategies for ARDS patients. Also, the study did not evaluate the effects of fluid balance and sedation depth on survival and respiratory mechanics. Changes in fluid balance may directly influence the degree of pulmonary edema, thereby affecting oxygenation and the assessment of MP. Excessive fluid accumulation can exacerbate pulmonary edema, reducing oxygenation efficiency, while inadequate fluid balance may impair circulation and compromise systemic organ function. Sedation depth is also a critical variable. Insufficient sedation may increase spontaneous breathing efforts, disrupting synchrony with mechanical ventilation and impairing its effectiveness. Conversely, excessive sedation can suppress spontaneous breathing, potentially affecting hemodynamic stability. Future studies should seek to incorporate assessments of fluid balance and sedation depth to better understand their roles in optimizing mechanical ventilation strategies.
Additionally, this study did not specifically compare the effects of different positions on thoracic compliance, nor did it report complications associated with prone positioning, such as pressure ulcers, facial edema, or nerve injuries. These complications can significantly influence patient outcomes during prolonged prone positioning. Future research should investigate the effects of these factors on ARDS patients. Future studies should also seek to increase the sample sizes and extend the follow-up periods. A comprehensive evaluation of the safety and efficacy of prone and lateral-prone positions is essential. Assessing the incidence of prone-related complications could provide valuable insights into refining lung-protective ventilation strategies, facilitating their better application in clinical practice, reducing the occurrence of VILI, and ultimately improving ARDS patients’ survival rates and quality of life.
Conclusions
Compared to prone ventilation, lateral-prone ventilation significantly reduced MP in ARDS patients. The early adoption of lateral-prone ventilation may have the potential to be popularized in clinical application and is worthy of further verification and optimization.
Acknowledgments
None.
Footnote
Reporting Checklist: The authors have completed the TREND reporting checklist. Available at https://jtd.amegroups.com/article/view/10.21037/jtd-2025-267/rc
Data Sharing Statement: Available at https://jtd.amegroups.com/article/view/10.21037/jtd-2025-267/dss
Peer Review File: Available at https://jtd.amegroups.com/article/view/10.21037/jtd-2025-267/prf
Funding: None.
Conflicts of Interest: All authors have completed the ICMJE uniform disclosure form (available at https://jtd.amegroups.com/article/view/10.21037/jtd-2025-267/coif). The authors have no conflicts of interest to declare.
Ethical Statement: The authors are accountable for all aspects of the work in ensuring that questions related to the accuracy or integrity of any part of the work are appropriately investigated and resolved. This study was conducted in accordance with the Declaration of Helsinki and its subsequent amendments. The study was approved by the Ethics Committee of Lishui Central Hospital (ethics approval No. 2021-311) and informed consent was taken from the patients or their legal guardians.
Open Access Statement: This is an Open Access article distributed in accordance with the Creative Commons Attribution-NonCommercial-NoDerivs 4.0 International License (CC BY-NC-ND 4.0), which permits the non-commercial replication and distribution of the article with the strict proviso that no changes or edits are made and the original work is properly cited (including links to both the formal publication through the relevant DOI and the license). See: https://creativecommons.org/licenses/by-nc-nd/4.0/.
References
- Bellani G, Laffey JG, Pham T, et al. Epidemiology, Patterns of Care, and Mortality for Patients With Acute Respiratory Distress Syndrome in Intensive Care Units in 50 Countries. JAMA 2016;315:788-800. [Crossref] [PubMed]
- Yang X, Yu Y, Xu J, et al. Clinical course and outcomes of critically ill patients with SARS-CoV-2 pneumonia in Wuhan, China: a single-centered, retrospective, observational study. Lancet Respir Med 2020;8:475-81. [Crossref] [PubMed]
- Abrams D, Schmidt M, Pham T, et al. Mechanical Ventilation for Acute Respiratory Distress Syndrome during Extracorporeal Life Support. Research and Practice. Am J Respir Crit Care Med 2020;201:514-25. [Crossref] [PubMed]
- Chen L, Xia HF, Shang Y, et al. Molecular Mechanisms of Ventilator-Induced Lung Injury. Chin Med J (Engl) 2018;131:1225-31. [Crossref] [PubMed]
- Patterson TJ, Currie P, Williams M, et al. Ocular Injury Associated With Prone Positioning in Adult Critical Care: A Systematic Review and Meta-Analysis. Am J Ophthalmol 2021;227:66-73. [Crossref] [PubMed]
- Santos A, Motta-Ribeiro GC, de Prost N, et al. Regional pulmonary perfusion, blood volume, and their relationship change in experimental early ARDS. Sci Rep 2024;14:5832. [Crossref] [PubMed]
- He J, Liu Y, Li L, et al. Effect of different durations of prone ventilation on the efficacy of patients with acute respiratory distress syndrome: a small Meta-analysis. Zhonghua Wei Zhong Bing Ji Jiu Yi Xue 2024;36:508-13. [Crossref] [PubMed]
- Weiss TT, Cerda F, Scott JB, et al. Prone positioning for patients intubated for severe acute respiratory distress syndrome (ARDS) secondary to COVID-19: a retrospective observational cohort study. Br J Anaesth 2021;126:48-55. [Crossref] [PubMed]
- Schaller SJ, Scheffenbichler FT, Bein T, et al. Guideline on positioning and early mobilisation in the critically ill by an expert panel. Intensive Care Med 2024;50:1211-27. [Crossref] [PubMed]
- Venkatesan P. Updated ESICM guidelines on ARDS. Lancet Respir Med 2024;12:16-8. [Crossref] [PubMed]
- Matthay MA, Arabi Y, Arroliga AC, et al. A New Global Definition of Acute Respiratory Distress Syndrome. Am J Respir Crit Care Med 2024;209:37-47. [Crossref] [PubMed]
- Slutsky AS, Ranieri VM. Ventilator-induced lung injury. N Engl J Med 2013;369:2126-36. [Crossref] [PubMed]
- Bhurayanontachai R. Mechanical ventilator support and prone positioning in COVID-19 related pneumonia: mechanical ventilator support in COVID-19. Clin Crit Care 2021;29:e0004.
- Touchon F, Trigui Y, Prud'homme E, et al. Awake prone positioning for hypoxaemic respiratory failure: past, COVID-19 and perspectives. Eur Respir Rev 2021;30:210022. [Crossref] [PubMed]
- Simioli F, Annunziata A, Langella G, et al. Early Prone Positioning and Non-Invasive Ventilation in a Critical COVID-19 Subset. A Single Centre Experience in Southern Italy. Turk Thorac J 2021;22:57-61. [Crossref] [PubMed]
- Khan S, Choudry E, Mahmood SU, et al. Awake Proning: A Necessary Evil During the COVID-19 Pandemic. Cureus 2020;12:e8989. [Crossref] [PubMed]
- Gattinoni L, Collino F, Camporota L. Mechanical power: meaning, uses and limitations. Intensive Care Med 2023;49:465-7. [Crossref] [PubMed]
- Boesing C, Graf PT, Schmitt F, et al. Effects of different positive end-expiratory pressure titration strategies during prone positioning in patients with acute respiratory distress syndrome: a prospective interventional study. Crit Care 2022;26:82. [Crossref] [PubMed]
- Boesing C, Rocco PRM, Luecke T, et al. Positive end-expiratory pressure management in patients with severe ARDS: implications of prone positioning and extracorporeal membrane oxygenation. Crit Care 2024;28:277. [Crossref] [PubMed]
(English Language Editor: L. Huleatt)