Biocompatibility of a novel lung sealant based on functionalized polyoxazolines in an ovine model of parenchymal lung injury
Highlight box
Key findings
• Application of a novel gelatin sealing patch impregnated with N-hydroxysuccinimide ester functionalized poly(2)oxazolines (NHS-POx) to superficial lung injuries in an ovine model causes a similar inflammatory response at 5 days and 2 weeks as compared with a fibrin patch (TachoSil®). At 2 weeks, healing with mesothelial coverage occurs and with localized visceral and parietal pleural thickening at 6 weeks. At 6 weeks, it is highly likely that the novel patch is completely degraded, without a significant residual inflammatory response.
What is known and what is new?
• Prolonged pulmonary air leakage (PAL) is associated with a more adverse post-operative course, and there is an unmet clinical need for more effective lung sealants. The NHS-POx patch was previously shown to be effective for lung sealing in ex-vivo and in-vivo models of short-term PAL.
• The findings of the present manuscript point towards comparable to favorable local biocompatibility properties, compared to the fibrin patch (TachoSil®).
What is the implication, and what should change now?
• The NHS-POx patch should be studied on diseased lungs (e.g., emphysema) with prolonged air leakage, to investigate the long-term effectiveness for air sealing. Additional safety studies are recommended, including impact on adhesions, post-operative infections, and pulmonary function.
Introduction
Lung resections can result in pulmonary air leakage (PAL) after surgery, especially in patients with diseased lungs, such as in emphysema (1,2). Prolonged PAL (pPAL), defined as PAL lasting longer than 5 days, occurs in up to 30% of patients (1). pPAL is associated with a more adverse postoperative course, including increased risk of infections [e.g., empyema: odds ratio (OR) =8.5], re-admissions (OR =2), re-interventions (4.8%), and a higher mortality (OR =1.9) (3-8).
A potential solution to pPAL is the intraoperative application of lung sealants, but their routine use is not recommended (9,10). There is some evidence for potential benefits, for example, a reduction in hospital stay of ~2 days has been shown in a meta-analysis for a fibrin-thrombin patch (fibrin patch, TachoSil®) (11). However, there are still improvements to be made with regard to adhesive strength and efficacy. As an example, the average length of stay increase is 4 days in patients with pPAL, indicating a possible gap in effectiveness (8). Another downside is the use of humane fibrin and thrombin, which is a costly process that requires plasma donors. So, there currently is an unmet clinical need for a more effective lung sealant, preferably based on synthetic components that are easy to produce, with a favorable biodegradation profile while not causing an excessive immune response (12).
A novel bio-adhesive patch, based on a porcine gelatin carrier impregnated with N-hydroxysuccinimide ester functionalized poly(2)oxazolines (NHS-POx) and nucleophilically activated polyoxaxolines (NU-POx), might be promising (NHS-POx patch, GATT-Technologies B.V., Nijmegen, The Netherlands) (13-15). Initial preclinical investigations have demonstrated effective use on liver bleedings, and the patch has also been investigated in clinical trials for this indication (ClinicalTrials.gov identifier: NCT04819945 and NCT05385952) (16,17). The mechanism of action is based on covalent bonds between NHS-POx and tissue amines, crosslinking of NHS-POx with amines within the gelatin carrier and NU-POx, and synergistic action with coagulation mechanisms due to blood uptake (13,15). Market approval for Europe has been obtained in 2023 for hemostatic use (marketed as ETHIZIA™, Ethicon Inc., Raritan, NJ, USA).
Our research group has previously tested the effectiveness of the NHS-POx patch for lung sealing purposes in an ex-vivo porcine lung model, showing superior aerostatic properties compared to commercially available sealants such as the fibrin-thrombin patch (TachoSil®) and favorable mechanical properties (18). Further investigations confirmed the sealing capabilities in a translational animal model of large air leaks (>400 mL/min) (19). Therefore, the NHS-POx patch might be a promising solution to the problem of pPAL.
However, the specific safety profile for intra-thoracic application of the NHS-POx patch, in terms of biodegradation and inflammation, is unknown. Previous safety experiments in a rat model have shown that the NHS-POx polymer degradation products are renally excretable (20). Experiments in a porcine model of intra-abdominal application showed complete degradation of NHS-POx impregnated gelatin patches in 4–6 weeks, with comparable histological response to control patches (including TachoSil®) (16).
The aim of the present study will be to assess the biocompatibility and safety characteristics of the novel NHS-POx patch for lung sealing applications, compared to a clinically used control patch (fibrin patch, TachoSil®), by examining local tissue inflammatory (foreign body) response, healing, and material biodegradation. We present this article in accordance with the ARRIVE reporting checklist (available at https://jtd.amegroups.com/article/view/10.21037/jtd-24-1733/rc).
Methods
Study design
Experimental layout
A translational large animal (ovine) model of superficial parenchymal lung injury was used, comparing the novel NHS-POx patch to a positive control group (fibrin patch, TachoSil®, Takeda Pharmaceutical Company Limited, Tokyo, Japan) and an untreated negative control group. Animals were sacrificed at 5 days, 2 weeks, and 6 weeks. Primary outcomes include histological response, healing, and material biodegradation. Secondary outcomes include adhesion formation, material migration, and adverse events. Three groups are tested per animal, ensuring each group is applied at least once on each predefined injury location per survival term. Researchers are blinded to group allocation until the moment of biomaterial application. A sample size of four per group per survival term is chosen for feasibility purposes, resulting in a total of 36 lesions in 12 sheep (Figure 1).
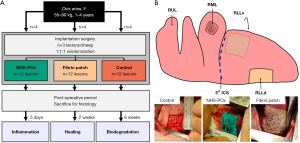
Lung lesions and biomaterial application
Standardized lesions are created in the same order: the right middle lobe (RML), right lower lobe ventral aspect (RLLv), and right lower lobe dorsal aspect (RLLd) (Figure 1). At a constant inflation pressure of 10 cmH2O, a 25 mm × 25 mm square is cut using a scalpel (#15) limited to 3 mm depth in a clamp, followed by visceral pleura removal and an additional 6×6 perpendicular cuts in this square. Baseline air leakage [Macchiarini scale, minimal leakage pressure (MLP)] and bleeding [surface bleeding severity scale (SBSS)] are recorded, and hemostasis is reached with gauze compression (21-23). For the NHS-POx patch (5 cm × 5 cm), the lung is primed using phosphate-buffered saline solution, followed by applying pressure with saline-wetted gauzes for 30 s, removal of the gauzes and reapplying pressure for another 90 s. The fibrin patch is applied using saline-wetted gauzes for 3 min. The lung is kept at 5 cmH2O static pressure during patch application, but ventilation is resumed if required.
Based on prior experiments, it is known that the lesions do not result in PAL due to intrinsic sealing, which facilitates the use of untreated lesions to compare the pathological mechanisms without necessitating prolonged chest drainage (21). Therefore, the current model is only capable of assessing biocompatibility and safety outcomes and not air leak-related outcomes.
Animal procedures
Ethical statement
All animal experiments were performed under a project license granted by national authorities after review by an independent ethics board [Radboud University Medical Center’s Dierexperimentencommissie (DEC), CCD nr. AVD10300 2021 14869]. Protocols were approved by the animal welfare body and registered at Radboud University Medical Center, Central Animal Laboratory (protocol nr. 2021-0012-003), in accordance with relevant national and international legislation (EU Directive 2010/63/EU).
Animals and housing
Adult female domestic sheep (Ovis aries, weight 55–90 kg, age 23–52 months) are hoof trimmed, sheared, treated with Spotinor Pour-On (to prevent ectoparasites) and housed in standard laboratory pens with a thick layer of sawdust bedding (4.5 m × 2.5 m for two animals, ambient temperature ~18–21 ℃, 12 h light/dark cycles). They are fed hay ad libitum and have continuous access to drinking water, and are additionally fed standard pellets. During housing and transportation, animals are always accompanied by at least one buddy sheep. Animals are transported to the laboratory at least 1 day before surgery, and in the 2- and 6-week groups, transported back to the farm at least 5 days post-operatively, and housed with a flock of sheep until being transported back for sacrifice. The housing conditions at the farm included a large stable (12 m × 5 m, hay bedding, large open doors and natural ventilation, willow branches as cage enrichment), ad libitum hay and water, and are fed additionally pellets, beet pulp, and mineral lick. Female sheep were chosen because they are not aggressive when housed in groups, and no relevant influences of sex are expected on biocompatibility outcome measures.
After histologically encountering a parasitic lung infection following the first two experiments, the remaining animals were separated from the larger flock to a clean stable and treated prophylactically with ivermectin subcutaneously (20 mg).
Anesthesia, analgesia, and antibiotic prophylaxis
Pre-medication included midazolam (0.7 mg/kg) and ketamine (10 mg/kg) intramuscularly. Anesthesia was induced using propofol (2 mg/kg), methadone (0.2 mg/kg), and ketamine (1 mg/kg) intravenously, and maintained using propofol, ketamine (0.2 mg/kg/h), remifentanil (0.06 mg/kg/h), and isoflurane. Doses were titrated to maintain the mean arterial pressure between 50 and 100 mmHg. Antibiotic prophylaxis included amoxicillin (10 mg/kg) intravenously 30 min before incision, and ampicillin (15 mg/kg) intramuscularly after surgery and again after 48 hours. Multi-modal analgesia included meloxicam (0.5 mg/kg) intramuscularly during induction and daily for the first 5 days postoperatively (orally 0.4 mg/kg), magnesium sulphate (40 mg/kg) intravenously during surgery, fentanyl (100 µg/h) applied trans-dermally during induction and an intercostal block at three levels using ropivacaine (1.5 mg/kg). In case of discomfort post-operatively, additional ketamine (0.5 mg/kg) was administered intramuscularly (Table S1). In the first animal, a slightly different variant of this anesthesia protocol was used (Table S2).
Surgical procedures
Animals were shaven, washed with soap containing chlorhexidine gluconate (40 mg/mL, HiBiSCRUB®) and disinfected using povidone-iodine solution (100 mg/mL, Betadine®). Blood pressure was measured using a percutaneous or surgically placed intra-arterial line in the groin. Surgery was performed by a board-certified surgeon (R.P.G.t.B.). After sterile draping, an incision was made in the right hemithorax in the fifth intercostal space through skin, subcutaneous muscle and subcutaneous fat. The latissimus dorsi muscle was split in the direction of the muscle fibers and the serratus anterior muscle was mobilized (muscle sparing approach). The thorax was entered in the fifth intercostal space and two perpendicular retractors were used for exposure. After experimental procedures, airtight closure was obtained in layers: transcostal sutures (2 Vicryl), latissimus dorsi (0 Vicryl), subcutaneous muscle (2-0 Vicryl), and skin (4-0 Monocryl) (24). A silicone chest tube was placed apically in the thorax (size Ch30). The anesthetic depth was lowered to allow for spontaneous ventilation, and the chest tube was removed when there was no relevant air leak (<10–20 mL/min), as assessed by digital chest drainage (Thopaz®, Medela, Baar, Switzerland). Wounds were sprayed with chlortetracycline (CTC) hydrochloride [2.45% weight in weight (w/w), CTC spray] for prevention of wound-infections and the sheep was allowed to recover in the pen.
Post-operative monitoring and euthanasia
Post-operatively, animals are checked using welfare monitoring lists, by scoring activity, social interaction, fur, dehydration, breathing, nose, eyes, feces, movement/gait, grimace, posture, eating/drinking and wound healing (0: normal, 1: slightly abnormal, 2: abnormal, max 26 points total). In case of aberrant breathing, lung auscultation is performed in combination with pulse oximetry to assess for a significant pneumothorax. For euthanasia at different timepoints, animals are administered ketamine and midazolam for anxiolysis, followed by an overdose of pentobarbital intravenously. Humane endpoints were defined in the institutional protocol and included severe weight loss (>20%), fever or infections (>3 days), apathic behavior (>1 day), diminished food or fluid intake (1–2 days), changes in defecation (>3 days), aberrant breathing (>3 days), prolonged air leak (>5 days) or pneumothorax and prolonged need for analgetic medication (>7 days).
Post-mortem procedures
Obduction and macroscopy
Obduction is performed through a median sternotomy, with right subcostal extension for exposure of all lesions. Macroscopic findings are systematically recorded and photographed. Presence of biomaterial migration is inspected before and after manipulation of the lung. Adhesions of the lesions are graded using the Zühlke classification (scale 0–4) (25). Any other adverse findings are recorded. Pictures and histology were taken after lung explantation or in-situ after further incision extension, as determined ad hoc by the method that would results in the least risk of manipulating the tissue around the lesions.
Histology: sampling, processing, and scoring
Histology samples are taken for systematic scoring: two per sealant (centrally through lesion and with healthy pleural overlap), one centrally through lesion for negative control (Figure S1). Additional samples were taken for qualitative analysis, including sections of unaffected lung tissue, parietal pleura, lymph nodes, and other macroscopically relevant lesions. All samples were stored in 4% formaldehyde, embedded in paraffin, sliced in 4-µm thick sections, and stained with hematoxylin-eosin (H&E). Slices were digitalized using the Pannoramic 1000 (3DHISTECH, Budapest, Hungary) and assessed using CaseViewer software (3DHISTECH, version 2.4).
Sections for systematic scoring were assessed in a randomized and blinded fashion by a researcher (B.P.H.) and a board-certified pathologist (S.V.), and consensus was reached after independent grading. In or directly adjacent to the pleura and biomaterial, cell response (polymorphonuclear cells, lymphocytes, plasma cells, macrophages, giant cells, necrosis) and biomaterial response (neovascularization, fibrosis, fatty infiltrate) were graded semi-quantitatively (scale 0–4) (Table S3, based on ISO 10993-6:2016) (26). Total response is calculated as the sum of cell response and biomaterial response. Healing was graded according to the Shafer criteria (1: very light healing; 2: moderate healing; 3: advanced healing; 4: well-organized) (27). Biodegradation was assessed semi-quantitatively (on central slides and macroscopic photos), estimating the proportion of the patch remaining at the application site (0: no material remaining; 1: 1–10%; 2: 11–25%; 3: 26–50%; 4: 51–75%; 5: 76–100%) in comparison to control samples of both patches right after application. Qualitative pathological descriptions were obtained.
Due to the unexpected encounter of chronic granulomatous inflammation due to a parasitic infection in the lung parenchyma, additional scoring was obtained to assess impact on the primary study outcomes. These were assessed in the lung parenchyma and included: granulomatous inflammation and associated presence of eosinophils and parasites, smallest distance from granuloma to pleura (mm), and eosinophilia at the pleural interface (scale 0–4: absent, rare, minimal, moderate, packed). Granulomas were sometimes located right below the pleura. In these cases, the inflammatory response associated with this granulomatous reaction was not counted towards the protocolar histological scoring, which was possible due to clear histological distinguishability (Figure S2).
Statistical analysis
Primary outcome measures, including histological (cell response, biomaterial response, total response), healing, and biodegradation scores, are compared between groups within each survival term. Histological scores are compared between central and pleural overlap slides within sealant groups, while all comparisons between groups are made based on central histological slides. Relevant qualitative findings in the pathological descriptions are counted and presented as frequencies. All animals that survived until the follow-up period were included in the analysis. Intra-thoracic complications leading to a humane endpoint and confounding the outcome parameters (e.g., empyema, pneumothorax, pneumonia) were considered as exclusion criteria.
Dichotomous data are presented as frequencies and comparisons are made using Fisher’s exact test (between animal comparisons) or Cochran’s Q test (within-animal comparisons between groups). Interval or continuous data is presented as median and range and comparisons are made using Kruskal-Wallis test (between animal comparisons) or Friedman’s test (within-animal comparisons between groups). Tests are performed two-tailed at an alpha of 0.05. A closed testing procedure is used for pairwise comparisons, so pairwise comparisons are only performed in case the overall test for the three groups is significant. Pairwise comparisons are performed using a Wilcoxon signed-rank test at an alpha of 0.05. Statistical testing is performed using SPSS (version 29; IBM Corp., Armonk, NY, USA).
Post-hoc analysis
Because residual patch material in a sample from the NHS-POx group at 6 weeks showed typical histological resemblance to the fibrin patch, additional post-hoc analysis (after blinded grading) was performed to identify whether an accidental mix-up could have occurred based on: (I) analysis of notes taken during experiment and photos taken during obduction; (II) additional staining based on ‘Elastin according to Masson (EvM)’ staining, resulting in blue/green staining of collagen fibers such as in the fibrin patch; and (III) blinded histological sample grouping task by an independent pathologist not related to the study project based on H&E and EvM staining. Results from this analysis were combined in an ‘adjudication report’ which was also assessed by an independent surgeon not related to the study project.
Results
General observations: surgery, post-operative course, obduction
The surgical procedure was successful in all twelve animals, creating a total of 36 lesions. Characteristics were similar over all time points, except weight gain and estimated operative blood loss (Table 1). In two animals (5-day and 6-week groups), dense adhesions were found during surgery, resulting in iatrogenic lung lacerations during mobilization that were sutured (Table S4). Lesions produced similar baseline bleeding and air leaks across groups, but air leak varied significantly based on lesion location (Tables S5,S6). Chest tubes were removed in the operating room and post-operative recovery was prosperous (Table 1). All animals survived the follow-up period, and there were no major complications that were attributed to the tested biomaterials. All samples were included in the analysis. Several complications that did not require additional interventions were noted (Table 1 and Table S7). Other minor protocol deviations are described in Table S4.
Table 1
Baseline characteristics | 5 days (n=4) | 14 days (n=4) | 42 days (n=4) | P value |
---|---|---|---|---|
Procedure characteristics | ||||
Weight (kg) | ||||
Baseline | 74.6 [56.3–87.6] | 76.6 [61.3–89.4] | 71.5 [67.5–79] | 0.78 |
Obduction | 73.6 [55.8–85.5] | 78.2 [62–89.4] | 77.6 [71.9–78.8] | 0.78 |
Change | −1.0 [−2.1 to −0.5] | 0.9 [0–2.3] | 4.7 [−0.2 to 7.4] | 0.02* |
Age (months) | 37 [23–48] | 33 [25–51] | 38 [28–52] | 0.87 |
Disease symptoms† | >0.99 | |||
Any | 2 [50] | 2 [50] | 2 [50] | |
Lung related | 0 [0] | 0 [0] | 0 [0] | |
Surgery characteristics | ||||
Surgery duration (min) | 182 [152–193] | 128 [116–160] | 129 [117–257] | 0.16 |
Pmax (cmH2O) | 28 [20–31] | 20 [20–22] | 21 [20–22] | 0.14 |
Estimated blood loss (mL)‡ | 250 [50–300] | 15 [10–20] | 70 [50–350] | 0.02* |
Rib fracture | 2 [50] | 1 [25] | 1 [25] | >0.99 |
Iatrogenic lung laceration | 1 [25] | 0 [0] | 1 [25] | >0.99 |
Physiological values | ||||
Systolic blood pressure (mmHg) | 101 [89–126] | 118 [100–128] | 102 [93–122] | 0.40 |
Baseline ACT (s) | 138 [95–161] | 125 [113–155] | 146 [133–173] | 0.44 |
pH | 7.35 [7.33–7.51] | 7.38 [7.35–7.43] | 7.38 [7.36–7.40] | 0.60 |
Lactate (mmol/L) | 2.1 [0.9–2.7] | 1.5 [1.4–2.7] | 1.8 [1.6–3.2] | 0.44 |
HCO3− (mmol/L) | 28.8 [24.2–32.7] | 29.9 [29.1–30.5] | 27.4 [26.7–30.4] | 0.62 |
Intra-operative findings | ||||
Adhesions | 1 [25] | 0 [0] | 1 [25] | >0.99 |
Infiltrates | 1 [25] | 0 [0] | 2 [50] | 0.71 |
Biopsied | 0 [0] | 0 [0] | 2 [50] | 0.27 |
Post-operative | ||||
Welfare score | ||||
Day 0 | 3 [0–5.5] | 1.5 [1–2] | 1.3 [0.5–2] | 0.92 |
Day 1 | 0.6 [0–1] | 1 [0.5–2] | 0.3 [0–0.5] | 0.07 |
Day 2 | 0 [0–1] | 0 [0–1] | 0 [0–1] | 0.96 |
Adverse events | ||||
No complication | 2 [50] | 2 [50] | 3 [75] | >0.99 |
Any deviation, no interventions§ | 2 [50] | 2 [50] | 1 [25] | >0.99 |
General obduction | ||||
Macroscopic infiltrates | ||||
Any | 3 [75] | 1 [25] | 4 [100] | 0.20 |
Under patch | 1 [25] | 0 [0] | 0 [0] | >0.99 |
Pleural effusion | 0.46 | |||
Serous | 1 [25] | 3 [75] | 3 [75] | |
Serosanguinous | 3 [75] | 1 [25] | 1 [25] | |
Incisional adhesion | 4 [100] | 3 [75] | 2 [50] | 0.71 |
Data are presented as median [range] or n [%]. Statistical testing performed with Kruskall-Wallis test or Fischer exact test, two-sided with alpha =0.05. †, minor pre-operative symptoms, including mastitis, paw infection, asymptomatic swelling. ‡, gross estimate of total blood loss made by the operating surgeon. §, minor complications including elevated respiratory rate, groin hematoma, low appetite, seroma, thickened udder. In one sheep, a gauze was left behind in the thorax (found at obduction), without causing any clinical symptoms. This gauze was encapsulated and not directly related to any patch in the thorax. ACT, activated clotting time; Pmax, maximum ventilatory pressure applied during surgery.
Analysis of suspected mix-up
The results of the independent mix-up analysis of a sample in the 6-week group are shown in appendix available at https://cdn.amegroups.cn/static/public/jtd-24-1733-1.pdf, showing converging evidence that a mix-up occurred during biopsy and labeling. The mix-up has some consequences for the histological analysis, and no consequences for macroscopic outcomes. For the histological analysis both original and adapted data were analyzed. There were no noteworthy changes in statistical significances between original and adapted data for between-group comparisons, except lymphocytes and fatty infiltrate barely reaching significance (P=0.05) (Table S8). Descriptively, adapted data demonstrated a lower phagocyte response, chronic inflammation, and necrosis in NHS-POx, as a result of remnant patch material in the suspected mix-up group (Figures 2,3). In the adapted data, this remnant patch material is identified as fibrin patch instead of NHS-POx.
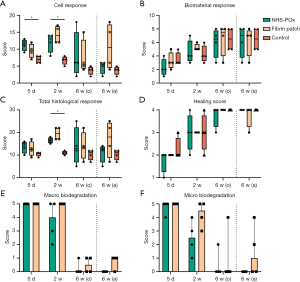

Histology: inflammation, healing, biodegradation
Within-group comparisons for central vs. pleural overlap slides revealed no significant differences (Table S9). Central slides are used for further between-group comparisons. Cell response was significantly higher for NHS-POx vs. control [score: 11.5 (range, 9–13) vs. 7 (range, 6–8), P=0.005] at 5 days, and for fibrin patch vs. control [score: 14 (range, 12–17) vs. 7 (range, 5–8), P=0.022] at 2 weeks. At 6 weeks, cell response was similar between groups (P=0.22), with outliers due to granulomatous chronic inflammation to residual patch material (n=1 fibrin patch and n=1 suspected mix-up sample likely fibrin patch). Biomaterial response scores were similar across groups. The total histological score was significantly higher for the fibrin patch vs. control group at 2 weeks [score: 19.5 (range, 17–22) vs. 11 (range, 10–12), P=0.008], but were similar at 42 days across groups (P=0.53) (Figure 2A-2C, Table S8).
Sub-analysis revealed similar patterns of classical wound healing, starting with acute inflammation, followed by chronic and phagocytotic inflammation and scar formation. Acute inflammation was similar across groups (polymorphonuclear cells, Figure 3A). Signs of chronic inflammation (lymphocytes and plasma cells) were higher in the fibrin patch group [score 6.5 (range, 5–7)] compared to NHS-POx [score: 3.5 (range, 3–5), P=0.03] and control [score: 3.5 (range, 3–4), P=0.03] at 2 weeks. At 6 weeks, adapted data showed higher chronic inflammation associated with necrotizing granulomatous reaction to remnant fibrin patch material, albeit not statistically significant (P=0.08, Figure 3B and Figure S3). Phagocyte response (macrophages and giant cells) was higher in the NHS-POx group [score: 4.5 (range, 4–6)] compared to control [score: 2.5 (range, 2–3), P=0.01] and fibrin patch [score: 3.5 (range, 2–4), P=0.08] at 5 days, while NHS-Pox [score: 5.5 (range, 4–7), P=0.03] and fibrin patch [score: 6 (range, 4–7), P=0.03] both had a significantly higher phagocyte response compared to control [score: 2.5 (range, 2–3)] at 14 days (Figure 3C). There was up to moderate necrosis at 2 weeks in both NHS-POx and fibrin patch groups (P=0.20), and up to severe necrosis in fibrin patch group (adapted data) at 6 weeks (P=0.14) (Figure 3D). Groups showed a similar increase in neovascularization and fibrosis across until 6 weeks (Figure 3E). Minimal fat was found associated with fibrosis in NHS-POx at 6 weeks (P=0.050), but this was also found in pleural overlap slides of the fibrin patch group at 6 weeks (Figure S4).
Healing scores were similar across groups (Figure 2D), showing overall well-organized healing at 6 weeks in both groups (P=0.37). At 2 weeks, fibrous and mesothelial coverage of the patch material could be observed (Figure 4A-4C). At 6 weeks, local pleural thickening could be seen at the prior patch location (Figure 4D-4F). Subpleural type II hyperplasia was noted at 5 days (Figure S3). Microscopically, based on adapted data, the NHS-POx patch was degraded at 6 weeks, while in two cases, there were microscopic remnants in the fibrin patch group with associated granulomatous reaction (Figure 5). Macrophages with a foamy aspect were observed adjacent degrading material in NHS-POx, and also within the pleura at 6 weeks in both NHS-POx and fibrin patch groups (Figure S3). Qualitative overview of histological findings is provided in appendix available at https://cdn.amegroups.cn/static/public/jtd-24-1733-2.docx.
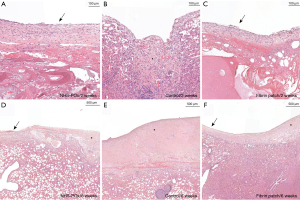
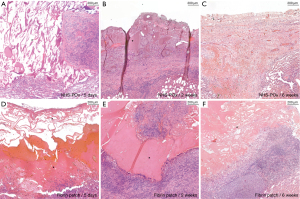
Additional polarized light microscopy in a sample of slides revealed no birefringence in the patch material or in the foamy macrophages. Birefringence was seen in a previously unidentified non-staining structure in an NHS-POx sample (adapted data) and in a giant cell in a fibrin patch sample at 6 weeks. Second look of a sample of lymph nodes revealed foamy macrophages in a lymph node at 6 weeks, with a single birefringent needle-like structure of unknown significance (Figure S5).
Macroscopy: obduction, adhesions, and migration
At obduction, pleural effusion was seen in all cases (estimated <150 mL), which appeared more serosanguinous at 5 days and more serous at 14 days and 6 weeks. There were adhesions between the incision and the lung in 9/12 (75%) animals, without relation to any of the lesions (Table 1). In the two animals with pre-existing adhesions during surgery, there also were extensive generalized adhesions during obduction, making adhesion grading difficult (Table S4). In one animal, an encapsulated gauze that was left behind during surgery was found. This gauze was used to control bleeding from extensive adhesiolysis to mobilize the lung during the initial procedure, at a site remote from any of the lesions. Another animal at 2 weeks showed marked pleural thickening.
Adhesions up to Zühlke grade 3 were found in the NHS-POx and untreated control groups, and up to grade 2 in the fibrin patch group (Figure 6A-6D). There were no significant differences between groups regarding adhesion presence or severity (Table S10). Migration of a small material segment was noted in 2/4 NHS-POx and 1/4 fibrin patch cases at 5 days without additional adverse findings, but these migrations were only observed after the lung had been manipulated during obduction. Macroscopically, based on the adapted data, NHS-POx patch showed no more remnants at 6 weeks, while 3/4 (75%) fibrin patch cases showed grade 1 remnants (estimated 1–10%) (P=0.08) (Figure 2E). Complete overview of macroscopic findings is provided in appendix available at https://cdn.amegroups.cn/static/public/jtd-24-1733-3.pdf.
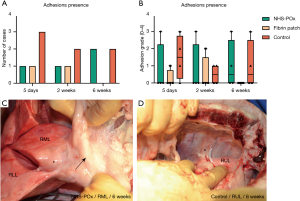
Qualitative histology: additional findings, pleural reaction, and chronic granulomatous inflammation
Additional qualitative analysis (Table S11) of unaffected lung and parietal pleura biopsies revealed signs of pleural irritation in 20/28 (71.4%) of samples at 2 weeks, but only in 3/27 (11.1%) at 6 weeks. In 12/27 (44.4%) of samples at 6 weeks, pleural thickening was seen, especially in samples across prior patches/lesions (Table 2). Histological signs of bronchopneumonia were noted in one animal at 2 weeks, but this animal was otherwise asymptomatic. Lymph nodes were unremarkable besides signs of chronic granulomatous inflammation due to parasitic infections.
Table 2
Histological response | 5 days | 14 days | 42 days |
---|---|---|---|
Pleural thickening | |||
Lung without lesion | 1/4 (75.0) | 4/4 (100.0) | 4/4 (100.0) |
Parietal pleura† | 0/9 (0.0) | 4/12 (33.3) | 1/12 (8.3) |
Across NHS-POx | N/A | 2/4 (50.0) | 2/4 (50.0) |
Across fibrin patch | N/A | 3/4 (75.0) | 2/3 (66.7) |
Across control lesion | N/A | 2/4 (50.0) | 3/4 (75.0) |
Total | 1/13 (7.7) | 15/28 (53.6) | 12/27 (44.4) |
Pleural irritation‡ | |||
Lung without lesion | 2/4 (50.0) | 3/4 (75.0) | 0/4 (0.0) |
Parietal pleura† | 3/9 (33.3) | 6/12 (50.0) | 0/12 (0.0) |
Across NHS-POx | N/A | 4/4 (100.0) | 0/4 (0.0) |
Across fibrin patch | N/A | 4/4 (100.0) | 1/3 (33.3) |
Across control lesion | N/A | 3/4 (75.0) | 2/4 (50.0) |
Total | 5/13 (38.5) | 20/28 (71.4) | 3/27 (11.1) |
Data are presented as number/total (%). †, apical, lateral, costodiaphragmatic recess samples. ‡, based on immune cell infiltration, fibrinoid pleuritis, reactive mesothelial cells, neovascularization. N/A, not available; NHS-POx, N-hydroxysuccinimide ester functionalized poly(2)oxazolines.
A range of granulomatous reactions (eosinophilic, necrotizing, calcified) was seen in the lung parenchyma or lymph nodes of all animals. In 5/12 (41.7%) of animals, parasites were also found to be associated with this reaction (Figures S2,S6). Veterinary consultation revealed the most suspected causative agent to be Muellerius capillaris. Animals were generally asymptotic. No typical infectious symptoms were recorded, possibly related symptoms were elevated respiratory rate and low appetite in 2/12 (16.7%) (Table S7). Macroscopic infiltrates were seen in 8/12 (66.7%) of animals at obduction, and in one case right below a fibrin patch, which were histologically confirmed to be a granulomatous inflammation. Biopsies taken in two animals at index surgery also confirmed the presence of this inflammation before patch application.
There were no statistically significant differences between distribution of granulomas and parasites between animals at different time points or between the pathological samples of the groups (Figure S6, Tables S12,S13). There was minimal eosinophilia associated with the biomaterial in the pleura, and this was not significantly different between groups. Minimal distance from the granuloma to pleura was not significantly different between groups (Figure S2).
Discussion
The biocompatibility and safety of the novel NHS-POx patch as a lung sealant were investigated in a long-term translational animal model up to 6 weeks. We demonstrate that complete degradation of the NHS-POx patch at 6 weeks is highly likely. Application causes an acute inflammatory and foreign body response with predominantly polymorphonuclear cells, macrophages, and giant cells at 5 and 14 days. At 6 weeks inflammation was comparable between NHS-POx and fibrin patch in the original data, in the adapted data no significant residual inflammation was seen at 6 weeks for NHS-POx. Healing is observed with mesothelial coverage at 14 days and localized visceral and parietal pleural thickening at 6 weeks. Overall, these findings point towards a favorable biocompatibility profile for the novel NHS-POx patch for lung sealant use.
Comparison to previous literature
In comparison to the fibrin patch (TachoSil®), the NHS-POx patch showed favorable biodegradability, with highly likely no remaining material or significant immune response at 6 weeks. The fibrin patch is a valid control group from a translational perspective, being the most studied lung sealant clinically (11). In our study, patch remnants were found in 2/4 (50%) of the fibrin patch group at 6 weeks (adapted data), with associated granulomatous inflammation. These findings are consistent with data from previous pre-clinical investigations, supporting the validity: the Food and Drug Administration described remnants up to 4 months, and the European Medicines Agency up to 20 weeks (28,29). Getman and colleagues had similar findings, applying TachoSil® to the rat lung, describing residual lymphocyte infiltrations adjacent the applied patch at 6 weeks, which resolved at 12 weeks with no more remaining patch material (30).
Biocompatibility of NHS-POx on a gelatin carrier patch was directly compared to TachoSil® in two previous animal studies. In a rat model of liver application, no clear differences in inflammatory cells were found at 7 days (15). In a porcine model of liver application, both patches degraded within 3–4 weeks, and the histological patterns were considered comparable, however, a comprehensive sub-analysis was not performed, examining individual cell types (16). In our study, albeit not statistically significant, the total histological response score showed a trend towards being higher in the fibrin patch group at 2 and 6 weeks (adapted data). At least, these findings indicate that the novel NHS-POx patch does not result in a more adverse local tissue response compared to the current gold standard lung sealant.
Mechanisms involved in biodegradation and healing
The NHS-POx patch was diffusely infiltrated with immune cells, indicating replacement of the prior biomaterial with extracellular matrix material, while the visceral surface of the patch was covered with a mesothelial lining. In the biodegradation process, the amide and thioester bonds formed by NHS-esters are hydrolyzed, while the porcine gelatin carrier is degraded by hydrolysis, matrix metalloproteases (gelatinases, collagenases), and phagocytosis (31-33). While the release and excretion pathways of the polyoxazoline polymers from the application site are unknown, they are renally cleared once in the bloodstream (20).
The specific release mechanism from the application site requires further study, as macrophages with a ‘foamy’ aspect were identified at 6 weeks with unknown contents, raising the question whether all polymers are excreted, or whether there is a degree of local retention in macrophage phagosomes. For example, such foamy macrophages were reported in a rat study testing poly-L-lactide implants, but these contained birefringent granules (34). In our study, no significant birefringence was noted in the foamy macrophages (except a single birefringent structure in a lymph node). Because the macrophages were also present in a fibrin patch sample, the precise causal relation is unclear.
The degradation mechanisms of the fibrin patch are well understood, as its composed entirely of endogenous components. Humane fibrinogen and thrombin form a fibrin cloth upon wet tissue contact, with an equine collagen carrier as structural support (29). These components are degraded by plasmin and collagenases, respectively (35,36). A previous document from the European Medicines Agency describes the degradation process as ‘layer by layer by absorptive granulation tissue and conversion into a pseudo-capsule’, which is similar to the observed degradation mechanism in our study (29). A chronic inflammatory response was observed in association with the fibrin patch in our study, with a high number of plasma cells. This could be indicative of a humoral immunity response to the xenogeneic components in the ovine species, but requires further investigation (37).
Model validity
Ovis aries is a realistic surgical application model for biomaterials with a high translational value, due to similar anatomy, respiratory physiology, and coagulation mechanisms (38-44). However, species-specific immune system differences should be considered. Pulmonary intravascular macrophages (PIMs) are present in up to 20% of the capillary endothelium in sheep lung, while only present in humans under specific conditions, and sheep lung house less alveolar macrophages (45,46). PIMs are thought to have a phagocytic function of blood borne particles, and also seem to play an important role in mediation of the immune response (46). But, the extent and impact of these cells on the current study findings is unknown.
Chronic granulomatous inflammation with associated parasites was found in the lung parenchyma and lymph nodes of the sheep in this study. Causative agents in The Netherlands include Muellerius capillaris, Protostrongylus rufescens, Protostrongylus brevispiculum, Neostrongylus linearis, and Cystocaulus ocreatus (47). These infections are generally asymptomatic and a high prevalence has been described (36.1–73%) (48,49). Histologically, chronic (eosinophilic) granulomatous pneumonia has been described, consistent with our findings (50-53). Ivermectin treatment was used after the first two sheep, but may be ineffective for complete eradication due to inhibited larval stages and the possibility of re-infestation, as seen in the sheep at 6 weeks (49).
Our findings do not support the notion that the biomaterial itself is the cause of (eosinophilic) granulomatous inflammation. We confirmed histologically its presence during the index surgery, observed a limited eosinophilic response within the pleura, and noted an apparently equal distribution of granulomas across histological sections which were associated with parasites in 41.7% of animals. There is no evident pathology consistent with our findings in previous clinical and preclinical literature. Other adverse histological reactions that have been described include destructive parenchymal fibrosis, abscesses with leukocytes and debris, and nonspecific bronchopneumonia in preclinical lung sealant studies (54-56). Clinical literature also documents eosinophilic reactions (like pleural effusion and eosinophilia) to fibrin glue (57-59), poly-glycolic acid, polyethylene glycol, and foreign body reactions/granulomas to previously implanted biomaterials (60-68).
Chronic infections may skew the immune response, but influences are considered limited due to equal distribution of granulomas, and the testing of each group per animal (69). The biomaterial immune response may be overestimated in case of granulomas proximal to the pleura, but was accounted in the grading process (Figure S2). Application of biomaterials may provide a favorable environment for infections, but no evident local increase in granulomas was seen in NHS-POx and fibrin patch samples compared to control across group, with exception of the fibrin patch sample at 5 days (Figure S6).
Limitations
The mix-up analysis to identify the contents of the remnant patch material in a sample at 6 weeks shows strong converging evidence based on additional staining, independent and blinded histological analysis and analysis of obduction photos that a mix-up in biopsy and labeling has occurred, accidentally labeling a fibrin patch sample as NHS-POx. Therefore, we think that adapting the data is justified as the collected converging evidence seems to indicate a near certainty probability. However, it should be acknowledged that there are no previously validated methods to distinguish the remnant material with absolute certainty.
The main limitation of this study is the small sample size, and lack of a priori power calculations on the primary outcomes. Therefore, the findings where no statistical differences (e.g., adhesions, adverse events) were encountered should be interpreted with caution, due to possible type II errors. Histological grading was performed using semi-quantitative scales, which may have some degree of inter-observer variability (e.g., difference between minimal and moderate classifications), but was accounted for using two assessors and consensus meetings, allowing for valid comparisons between groups at different timepoints. These scales have inherent statistical limitations, being less powerful for detection of differences as compared to continuous measures. Finally, while all assessments of primary outcome measures were performed blinded, there were evident histological differences between groups and survival terms which may have biased assessment.
Because the inter-subject variability was expected to be lower than the intra-subject variability, each animal was taken as its own control to lower the total sample size required. Some inter-subject variability was observed (lobe-specific differences in baseline leak capacity based on lesion locations as can be seen in Table S6), but this is likely negligible on biocompatibility-related outcome measures due to rapid intrinsic sealing mechanisms in the healthy-lung model (21). A downside to this approach is cross-over effects due to whole-pleura responses. For example, signs of generalized pleural irritation were seen at 14 days, but this could also be a consequence of the surgery itself. Finally, root-cause analysis of adverse events affecting the whole animal is difficult, but the experiment was not powered for this outcome measure.
Some limitations to macroscopic outcome measures should also be noted. Adhesion assessment of specific lesions was sometimes difficult, especially in cases of diffuse intra-thoracic adhesions (i.e., in the animals with extensive pre-existing adhesions), and the RLLd location was sometimes difficult to expose. Migration was only found in this study after lung manipulation during obduction, which may have confounded this outcome measure. Biodegradation was sometimes difficult to assess on the photos, especially at 6 weeks.
Recommendations for future research
Extensive diffuse adhesions found during obduction can be sign of a serious adverse event, but this seems due to pre-existing adhesions. However, further study is required to assess the impact of NHS-POx on adhesion formation, preferably in a study design without potential cross-over effects of different groups within the same pleural cavity.
The current study could not investigate air leak-related parameters due to intrinsic sealing (21). As was discussed in our previous study, there currently are no long-term air leak models, so histological healing may have to be used as a surrogate for long-term sealing (19). Further study is required into the sealing capabilities of the NHS-POx patch in relation to wound healing, in order to prevent pneumothorax as a safety endpoint, especially in emphysematous lungs (19,21).
This study only primarily looked at local biocompatibility parameters, and further study with a higher sample size is required to study systemic effects and adverse events, such as impact on incidence of post-operative infections (i.e., bacteriostatic properties of the patch in a realistic environment). Also, this study did not look at the impact of local pleural thickening on the pulmonary function, but should be further investigated. Finally, further investigations are required to confirm the findings of our study, and investigations into the local immunogenic effects can be further specified by looking at influences on pro- vs. anti-inflammatory cytokines and macrophages differentiation (M1/M2 subtypes) (70).
Conclusions
The novel NHS-POx patch applied to the lung shows comparable to favorable local biocompatibility properties compared to existing fibrin patch. Complete biodegradation and no significant residual tissue response at 6 weeks are highly likely. Further research is required to study the impact on aerostatic efficacy and adverse events.
Acknowledgments
We would like to thank the Radboud Animal Welfare Body and the designated veterinarian Manon van Hulzen for help designing the protocols, biotechnicians Alex Hanssen, Stefanie Schönfeld and Maikel School for their excellent support during experimental procedures and Conrad van den Broek and Tom Wijga for their caretaking of animals. We also want to thank all other personnel of the Radboudumc Animal Laboratory involved with the experiments. We want to thank Juul van Lin and Merve Sairoglu for their help with data-acquisition and histological processing. Finally, we want to thank Heike Grabsch for help with the pathological analysis as an independent pathologist and Tim Lubbers for independently checking the adjudication report (both affiliated with the Maastricht University Medical Center).
Footnote
Reporting Checklist: The authors have completed the ARRIVE reporting checklist. Available at https://jtd.amegroups.com/article/view/10.21037/jtd-24-1733/rc
Data Sharing Statement: Available at https://jtd.amegroups.com/article/view/10.21037/jtd-24-1733/dss
Peer Review File: Available at https://jtd.amegroups.com/article/view/10.21037/jtd-24-1733/prf
Funding: This work was supported by
Conflicts of Interest: All authors have completed the ICMJE uniform disclosure form (available at https://jtd.amegroups.com/article/view/10.21037/jtd-24-1733/coif). H.v.G. was a scientific advisor for GATT Technologies B.V. until 31 December 2021, but not in relation to lung sealing technology. B.P.H. received funding and study materials through the institution for conduction of the study from GATT Technologies B.V. E.H.F.M.v.d.H. reports the following conflicts, all outside the scope of this paper and all fees are paid to the institution: unrestricted research grants to the department from AstraZeneca Oncology, Pentax Medical, Philips, Johnson & Johnson, Intuitive, KWF (national cancer fund) and IMAGIO (Innovative Health Initiative—EU fund), consulting fees to the department from Johnson & Johnson, Intuitive and NLC, travel arrangements from Johnson & Johnson and Intuitive and loan equipment from Philips Medical, Intuitive and Pentax. Speaker fees to the department from Janssen-Cilag, Siemens, Pentax, Ethicon, Astra Zeneca, Intuitive and Philips, travel arrangements from Siemens; holder of US Patent 12.048.494 and other patents pending outside the scope of this paper. He is an Executive Board member of European Association for Bronchology and Interventional Pulmonology (EABIP, unpaid). R.P.G.t.B. reports a research grant from a Dutch/Belgian governmental funding agency not related to the present study. The other authors have no conflicts of interest to declare.
Ethical Statement: The authors are accountable for all aspects of the work in ensuring that questions related to the accuracy or integrity of any part of the work are appropriately investigated and resolved. All animal experiments were performed under a project license granted by national authorities after review by an independent ethics board [Radboud University Medical Center’s Dierexperimentencommissie (DEC), CCD nr. AVD10300 2021 14869]. Protocols were approved by the animal welfare body and registered at Radboud University Medical Center, Central Animal Laboratory (protocol nr. 2021-0012-003), in accordance with relevant national and international legislation (EU Directive 2010/63/EU).
Open Access Statement: This is an Open Access article distributed in accordance with the Creative Commons Attribution-NonCommercial-NoDerivs 4.0 International License (CC BY-NC-ND 4.0), which permits the non-commercial replication and distribution of the article with the strict proviso that no changes or edits are made and the original work is properly cited (including links to both the formal publication through the relevant DOI and the license). See: https://creativecommons.org/licenses/by-nc-nd/4.0/.
References
- Attaar A, Tam V, Nason KS. Risk Factors for Prolonged Air Leak After Pulmonary Resection: A Systematic Review and Meta-analysis. Ann Surg 2020;271:834-44. [Crossref] [PubMed]
- DeCamp MM, Blackstone EH, Naunheim KS, et al. Patient and surgical factors influencing air leak after lung volume reduction surgery: lessons learned from the National Emphysema Treatment Trial. Ann Thorac Surg 2006;82:197-206; discussion 206-7. [Crossref] [PubMed]
- Yoo A, Ghosh SK, Danker W, et al. Burden of air leak complications in thoracic surgery estimated using a national hospital billing database. Clinicoecon Outcomes Res 2017;9:373-83. [Crossref] [PubMed]
- Brunelli A, Xiume F, Al Refai M, et al. Air leaks after lobectomy increase the risk of empyema but not of cardiopulmonary complications: a case-matched analysis. Chest 2006;130:1150-6. [Crossref] [PubMed]
- Liang S, Ivanovic J, Gilbert S, et al. Quantifying the incidence and impact of postoperative prolonged alveolar air leak after pulmonary resection. J Thorac Cardiovasc Surg 2013;145:948-54. [Crossref] [PubMed]
- Attaar A, Luketich JD, Schuchert MJ, et al. Prolonged Air Leak After Pulmonary Resection Increases Risk of Noncardiac Complications, Readmission, and Delayed Hospital Discharge: A Propensity Score-adjusted Analysis. Ann Surg 2021;273:163-72. [Crossref] [PubMed]
- Ponholzer F, Ng C, Maier H, et al. Risk factors, complications and costs of prolonged air leak after video-assisted thoracoscopic surgery for primary lung cancer. J Thorac Dis 2023;15:866-77. [Crossref] [PubMed]
- Brunelli A, Chapman K, Pompili C, et al. Ninety-day hospital costs associated with prolonged air leak following lung resection. Interact Cardiovasc Thorac Surg 2020;31:507-12. [Crossref] [PubMed]
- Aprile V, Bacchin D, Calabrò F, et al. Intraoperative prevention and conservative management of postoperative prolonged air leak after lung resection: a systematic review. J Thorac Dis 2023;15:878-92. [Crossref] [PubMed]
- Belda-Sanchís J, Serra-Mitjans M, Iglesias Sentis M, et al. Surgical sealant for preventing air leaks after pulmonary resections in patients with lung cancer. Cochrane Database Syst Rev 2010;2010:CD003051. [Crossref] [PubMed]
- Zhou J, Lyu M, Pang L, et al. Efficiency and safety of TachoSil® in the treatment of postoperative air leakage following pulmonary surgery: a meta-analysis of randomized controlled trials. Jpn J Clin Oncol 2019;49:862-9. [Crossref] [PubMed]
- Brunelli A, Bölükbas S, Falcoz PE, et al. Exploring consensus for the optimal sealant use to prevent air leak following lung surgery: a modified Delphi survey from The European Society of Thoracic Surgeons. Eur J Cardiothorac Surg 2021;59:1265-71. [Crossref] [PubMed]
- Boerman MA, Roozen E, Sánchez-Fernández MJ, et al. Next Generation Hemostatic Materials Based on NHS-Ester Functionalized Poly(2-oxazoline)s. Biomacromolecules 2017;18:2529-38. [Crossref] [PubMed]
- Boerman MA, Van der Laan HL, Bender JCME, et al. Synthesis of pH- and thermoresponsive poly (2-n-propyl-2-oxazoline) based copolymers. J Polym Sci A Polym Chem 2016;54:1573-82.
- Roozen EA, Warlé MC, Lomme RMLM, et al. New polyoxazoline loaded patches for hemostasis in experimental liver resection. J Biomed Mater Res B Appl Biomater 2022;110:597-605. [Crossref] [PubMed]
- Roozen EA, Lomme RMLM, Calon NUB, et al. Efficacy of a novel polyoxazoline-based hemostatic patch in liver and spleen surgery. World J Emerg Surg 2023;18:19. [Crossref] [PubMed]
- de Wilt JHW, Verhoef C, de Boer MT, et al. Clinical Safety and Performance of GATT-Patch for Hemostasis in Minimal to Moderate Bleeding During Open Liver Surgery. J Surg Res 2024;298:316-24. [Crossref] [PubMed]
- Hermans BP, Li WWL, Roozen EA, et al. Sealing effectiveness of a novel NHS-POx based patch: experiments in a dynamic ex vivo porcine lung. J Thorac Dis 2023;15:3580-92. [Crossref] [PubMed]
- Hermans BP, Ten Broek RPG, Li WWL, et al. Proof-of-principle of a lung sealant based on functionalized polyoxazolines: experiments in an ovine acute aerostasis model. Interdiscip Cardiovasc Thorac Surg 2024;38:ivae113. [Crossref] [PubMed]
- Boerman MA, Roozen EA, Franssen GM, et al. Degradation and excretion of poly (2-oxazoline) based hemostatic materials. Materialia 2020;12:100763.
- Hermans BP, Li WWL, Roozen EA, et al. Intrinsic pulmonary sealing, its mechanisms and impact on validity and translational value of lung sealant studies: a pooled analysis of animal studies. J Thorac Dis 2023;15:4703-16. [Crossref] [PubMed]
- Ardehali A, Spotnitz WD, Hoffman RW, et al. Evaluation of the safety and efficacy of a new hemostatic powder using a quantitative surface bleeding severity scale. J Card Surg 2019;34:50-62. [Crossref] [PubMed]
- Macchiarini P, Wain J, Almy S, et al. Experimental and clinical evaluation of a new synthetic, absorbable sealant to reduce air leaks in thoracic operations. J Thorac Cardiovasc Surg 1999;117:751-8. [Crossref] [PubMed]
- Rooney MB, Mehl M, Monnet E. Intercostal thoracotomy closure: transcostal sutures as a less painful alternative to circumcostal suture placement. Vet Surg 2004;33:209-13. [Crossref] [PubMed]
- Zühlke HV, Lorenz EM, Straub EM, et al. Pathophysiology and classification of adhesions. Langenbecks Arch Chir Suppl II Verh Dtsch Ges Chir 1990;1009-16.
- International Organization for Standardization. Biological evaluation of medical devices. Part 6: Tests for local effects after implantation. ISO 10993-6:2016. 2009.
- Deyhimi P, Khademi H, Birang R, et al. Histological Evaluation of Wound Healing Process after Photodynamic Therapy of Rat Oral Mucosal Ulcer. J Dent (Shiraz) 2016;17:43-8.
- Food and Drug Administration. Filing of Final Pre-Clinical Review of STN 125351-0. TachoSil. Available online: https://www.fda.gov/vaccines-blood-biologics/approved-blood-products/tachosil
- European Medicines Agency (EMA). Scientific discussion for the approval of TachoSil. Available online: https://www.ema.europa.eu/en/medicines/human/EPAR/tachosil#ema-inpage-item-authorisation-details
- Getman V, Devyatko E, Wolner E, et al. Fleece bound sealing prevents pleural adhesions. Interact Cardiovasc Thorac Surg 2006;5:243-6. [Crossref] [PubMed]
- Rubin R, Strayer DS, Rubin E. Chapter 2: Inflammation. In: Rubin R, Strayer DS, Rubin E. Rubin's pathology: clinicopathologic foundations of medicine. 6th ed. Baltimore: Lippincott Williams & Wilkins; 2012.
- Nam S, Mooney D. Polymeric Tissue Adhesives. Chem Rev 2021;121:11336-84. [Crossref] [PubMed]
- Ullm S, Krüger A, Tondera C, et al. Biocompatibility and inflammatory response in vitro and in vivo to gelatin-based biomaterials with tailorable elastic properties. Biomaterials 2014;35:9755-66. [Crossref] [PubMed]
- De Jong WH, Eelco Bergsma J, Robinson JE, et al. Tissue response to partially in vitro predegraded poly-L-lactide implants. Biomaterials 2005;26:1781-91. [Crossref] [PubMed]
- Brown AC, Barker TH. Fibrin-based biomaterials: modulation of macroscopic properties through rational design at the molecular level. Acta Biomater 2014;10:1502-14. [Crossref] [PubMed]
- Helling AL, Tsekoura EK, Biggs M, et al. In Vitro Enzymatic Degradation of Tissue Grafts and Collagen Biomaterials by Matrix Metalloproteinases: Improving the Collagenase Assay. ACS Biomater Sci Eng 2017;3:1922-32. [Crossref] [PubMed]
- Massaro MS, Pálek R, Rosendorf J, et al. Decellularized xenogeneic scaffolds in transplantation and tissue engineering: Immunogenicity versus positive cell stimulation. Mater Sci Eng C Mater Biol Appl 2021;127:112203. [Crossref] [PubMed]
- Gray ME, Meehan J, Sullivan P, et al. Ovine Pulmonary Adenocarcinoma: A Unique Model to Improve Lung Cancer Research. Front Oncol 2019;9:335. [Crossref] [PubMed]
- Weisskopf M, Kron M, Giering T, et al. The sheep as a pre-clinical model for testing intra-aortic percutaneous mechanical circulatory support devices. Int J Artif Organs 2021;44:703-10. [Crossref] [PubMed]
- Zhou K, Niu S, Bianchi G, et al. Biocompatibility assessment of a long-term wearable artificial pump-lung in sheep. Artif Organs 2013;37:678-88. [Crossref] [PubMed]
- Scheerlinck JP, Snibson KJ, Bowles VM, et al. Biomedical applications of sheep models: from asthma to vaccines. Trends Biotechnol 2008;26:259-66. [Crossref] [PubMed]
- Hemingway A, Hemingway C. Respiration of sheep at thermoneutral temperature. Respir Physiol 1966;1:30-7. [Crossref] [PubMed]
- Wilhelmi MH, Tiede A, Teebken OE, et al. Ovine blood: establishment of a list of reference values relevant for blood coagulation in sheep. ASAIO J 2012;58:79-82. [Crossref] [PubMed]
- Elvin CM, Vuocolo T, Brownlee AG, et al. A highly elastic tissue sealant based on photopolymerised gelatin. Biomaterials 2010;31:8323-31. [Crossref] [PubMed]
- Bouljihad M, Leipold HW. An ultrastructural study of pulmonary bronchiolar and alveolar epithelium in sheep. Zentralbl Veterinarmed A 1994;41:573-86. [Crossref] [PubMed]
- Schneberger D, Aharonson-Raz K, Singh B. Pulmonary intravascular macrophages and lung health: what are we missing? Am J Physiol Lung Cell Mol Physiol 2012;302:L498-503. [Crossref] [PubMed]
- Royal GD. Verschillende wormsoorten bij schapen. Accessed 03-06-2023. Available online: https://www.gddiergezondheid.nl/nl/Diergezondheid/Management/Wormen/Schapen/Algemeen-maagdarmwormen/verschillende-wormsoorten
- Hanks JE, Campbell AJD, Larsen JWA. Severity and prevalence of small lungworm infection on three South Australian farms and associations with sheep carcass characteristics. Vet Parasitol 2021;296:109503. [Crossref] [PubMed]
- Panuska C. Lungworms of ruminants. Vet Clin North Am Food Anim Pract 2006;22:583-93. [Crossref] [PubMed]
- Beresford-Jones WP. Observations on Muellerius capillaris (Müller, 1889) Cameron, 1927: III.—Experimental Infection of Sheep. Res Vet Sci 1967;8:272-81.
- Berrag B, Rhalem A, Sahibi H, et al. Bronchoalveolar cellular responses of goats following infections with Muellerius capillaris (Protostrongylidae, Nematoda). Vet Immunol Immunopathol 1997;58:77-88. [Crossref] [PubMed]
- Mansfield LS, Gamble HR. Alveolar mastocytosis and eosinophilia in lambs with naturally acquired nematode infections of Protostrongylus rufescens and Haemonchus contortus. Vet Immunol Immunopathol 1995;49:251-62. [Crossref] [PubMed]
- Gulbahar MY, Davis WC, Yarim M, et al. Characterization of local immune response against lungworms in naturally infected sheep. Vet Parasitol 2009;160:272-8. [Crossref] [PubMed]
- Ennker IC, Ennker J, Schoon D, et al. Formaldehyde-free collagen glue in experimental lung gluing. Ann Thorac Surg 1994;57:1622-7. [Crossref] [PubMed]
- Daniel P, Wehner DW, Morgenstern R, et al. Ultrasonic sealing of the lung parenchyma after atypical resection. Z Exp Chir Transplant Kunstliche Organe 1987;20:117-21.
- Levashev IuN, Bobkov AG, Varlamov VV, et al. Experimental evaluation of different methods of intraoperative aerostasis in surgery of the lungs. Grud Serdechnososudistaia Khir 1990;63-6.
- Kawamoto N, Okita R, Hayashi M, et al. Suspected fibrin glue-induced acute eosinophilic pneumonia after pulmonary resection: A case report. Thorac Cancer 2021;12:2126-9. [Crossref] [PubMed]
- Kawamoto N, Okita R, Okada M, et al. Fibrin glue-induced eosinophilic pleural effusion after pulmonary resection: A case report. Int J Surg Case Rep 2021;85:106239. [Crossref] [PubMed]
- Kambayashi T, Suzuki T. Eosinophilic pleural effusion possibly induced by fibrin sealant. Kyobu Geka 2012;65:141-4.
- Miyahara E, Ueda D, Kawasaki Y, et al. Polyglycolic acid mesh for preventing post-thoracoscopic bullectomy recurrence. Surg Today 2021;51:971-7. [Crossref] [PubMed]
- Doğan R, Uysal S, Kumbasar U, et al. Can surgical adhesives may cause false positivity in follow-up positron emission tomography after lung cancer resection? Tuberk Toraks 2021;69:59-64. [Crossref] [PubMed]
- Kurian EM, Abu-Hijleh M, Lowrey TR, et al. Oxidized Regenerated Cellulose (Surgicel®) on Cytology/Histology. Acta Cytol 2022;66:556-9. [Crossref] [PubMed]
- Okazaki M, Sano Y, Mori Y, et al. Two cases of granuloma mimicking local recurrence after pulmonary segmentectomy. J Cardiothorac Surg 2020;15:7. [Crossref] [PubMed]
- Suemitsu R, Tokito T, Ichiki M, et al. Complication of bovine pericardial buttress: pulmonary pseudotumor. Asian Cardiovasc Thorac Ann 2011;19:64-5. [Crossref] [PubMed]
- Sawada T, Watanabe Y, Oura H, et al. Pulmonary staple granuloma mimicking lung cancer; report of a case. Kyobu Geka 2008;61:591-4.
- Badenes D, Pijuan L, Curull V, et al. A foreign body reaction to Surgicel(®) in a lymph node diagnosed by endobronchial ultrasound-guided transbronchial needle aspiration. Ann Thorac Med 2017;12:55-6. [Crossref] [PubMed]
- Yousem SA, Amin RM, Levy R, et al. Pulmonary pathologic alterations associated with biopsy inserted hydrogel plugs. Hum Pathol 2019;89:40-3. [Crossref] [PubMed]
- Butnor KJ, Bodolan AA, Bryant BRE, et al. Impact of Histopathologic Changes Induced by Polyethylene Glycol Hydrogel Pleural Sealants Used During Transthoracic Biopsy on Lung Cancer Resection Specimen Staging. Am J Surg Pathol 2020;44:490-4. [Crossref] [PubMed]
- Ouaissi A. Regulatory cells and immunosuppressive cytokines: parasite-derived factors induce immune polarization. J Biomed Biotechnol 2007;2007:94971. [Crossref] [PubMed]
- Chandorkar Y. K R, Basu B. The Foreign Body Response Demystified. ACS Biomater Sci Eng 2019;5:19-44. [Crossref] [PubMed]