Computed tomography imaging features in Stanford type-A aortic dissection predict in-hospital rupture
Highlight box
Key findings
• Arc length ≥130 mm and the centerline distance from the sinotubular junction to the celiac trunk ≥391 mm were risk factors for aortic rupture in Stanford type A aortic dissection patients.
What is known and what is new?
• Some biochemical indicators and clinical symptoms, as well as radiographic characteristics like aortic diameter, the aortic height index, and periaortic hematoma have been associated with increased risk of ruptures.
• Our study aims to extract and analyze additional quantitative variables from computed tomography angiography and reconstructed images that may be associated with aortic rupture.
What is the implication, and what should change now?
• Incorporating these quantitative imaging features into predictive models could improve risk stratification, allowing for earlier surgical intervention in patients at the highest risk of rupture.
Introduction
Background
Stanford type A aortic dissection (TAAD) is defined as a dissection involving the ascending aorta. The condition can quickly become life-threatening if the weakened aortic wall ruptures or the dissection extends into the coronary arteries. Immediate surgical intervention is typically recommended for patients diagnosed with TAAD to prevent fatal outcomes (1). However, some patients may not receive timely surgery due to delays in diagnosis, patient preferences, comorbidities, or advanced age. According to recent data from the International Registry of Acute Aortic Dissection, the mortality rate for patients with nonoperative acute type A aortic dissection is 23.7% within the first 48 h (2). The Japan Registry of Aortic Dissection highlights the real-world clinical setting for treating acute type A aortic dissection in Japan, where 16.6% of medically treated patients die in-hospital, with aortic rupture or redissection as the leading cause of death (3).
Rationale and knowledge gap
Early identification of high-risk individuals susceptible to aortic rupture in TAAD is highly desirable, as this could aid physicians and patients in making timely decisions, optimizing medical resources, and ultimately reducing mortality. Several researchers have sought to identify clinical or laboratory markers that could predict the risk of rupture in patients with TAAD. Biochemical indicators, such as elevated troponin and D-dimer levels, as well as clinical symptoms like shock and severe back pain, have been associated with an increased risk of rupture (4,5). While computed tomography angiography (CTA) provides detailed visualization of aortic lesions, few studies have thoroughly explored imaging-based morphological risk factors for rupture in patients with TAAD.
Objective
Wu et al. (6) identified several radiographic characteristics that significantly predict in-hospital rupture in patients with TAAD, including aortic diameter, the aortic height index (AHI; the ratio of maximum diameter to height), periaortic hematoma, pleural effusion, brachiocephalic artery involvement, renal artery involvement, and hemopericardium. Despite these findings, much remains unexplored in terms of imaging-based predictors. Our study aims to extract and analyze additional quantitative variables from CTA and reconstruct images that may be associated with aortic rupture, to develop clinically useful predictive tools for improving risk stratification and patient outcomes. We present this article in accordance with the STROBE reporting checklist (available at https://jtd.amegroups.com/article/view/10.21037/jtd-24-1702/rc).
Methods
Patient selection and data collection
We conducted a retrospective cross-sectional study of TAAD cases. From January 2020 to May 2021, 45 TAAD patients without surgical treatment in the Department of Cardiovascular Surgery of Xiamen Cardiovascular Hospital of Xiamen University were recruited for this study. A retrospective review of their medical records was performed. The study was conducted in accordance with the Declaration of Helsinki and its subsequent amendments. The study protocol was approved by the Ethics Committee of Xiamen Cardiovascular Hospital (No. 2023036) and informed consent was taken from all the patients or their families. After excluding 14 patients who did not undergo CTA imaging at Xiamen Cardiovascular Hospital and one patient whose quality of image was poor, a total of 30 participants were divided into the rupture group (n=14) and non-rupture group (n=16) (Figure 1). Data collected for the study included demographic information, medical history, clinical presentation, imaging findings (from CTA and echocardiography), and patient outcomes.
Definitions
Aortic rupture in TAAD was defined by the following clinical manifestations: sudden loss of consciousness, autonomous cessation of breathing, loss of pulse, and gradual cessation of heartbeat. Additionally, massive effusion in the pericardium, chest cavity, or abdominal cavity, detected via ultrasound examination, was also considered indicative of aortic rupture. We categorized the phases of TAAD based on the classification system by Booher et al. (7), which analyzed survival outcomes according to the location of the dissection entry point and treatment strategy from the International Registry of Acute Aortic Dissection registry. The periods identified were as follows: 0–24 h (hyperacute), 2–7 days (acute), 8–30 days (subacute), and >30 days (chronic).
Morphological characteristics of computed tomography (CT) imaging
CTA scans were performed within 2 h of admission; otherwise, values were considered missing. All imaging data were retrieved from the medical system of Xiamen Cadiovascular Hospital and transferred to an independent 3D server (iNtuition, TeraRecon, Inc., Fremont City, CA, USA). Standardized measurements were obtained for all indices using iNtuition software by an experienced cardiovascular radiologist who was blinded to patient outcomes. Variables hypothesized to predict in-hospital rupture included the false lumen length (FLL), the maximum diameter of the aorta (Dmax), arc length (AL) of the false lumen (FL), the curvature of the FL (radian), the number of branch arteries arising from FL and tears, and the aortic tortuosity index (ATI) (Figure 2).
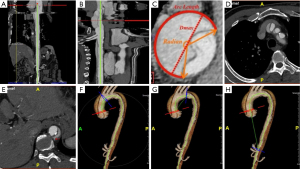
Statistical analysis
Statistical analyses were performed using SPSS version 21 (IBM Corp., Armonk, NY, USA). Continuous variables were expressed as either mean ± standard deviation (SD) or median with interquartile ranges, depending on the normality of the distribution. Missing values were replaced by either mean or median value of the variables dependent on their distribution. For comparison of continuous variables between two groups, the independent samples t-test was applied if the data were normally distributed. When normal distribution was not observed, the Mann-Whitney test was used. Categorical variables were presented as proportions and analyzed using Pearson’s Chi-square test or the Fisher-Irwin test, as appropriate. To identify risk factors associated with aortic rupture, significant variables were identified through single factor logistic regression. Receiver operating characteristic (ROC) curves were used to determine the cut-offs for significant variables. Odds ratios (ORs) and 95% confidence intervals (CIs) were calculated to assess the strength of the association. A P value of less than 0.05 was considered statistically significant.
Results
Among the 30 patients with nonoperative TAAD, 17 died in the hospital. Of these, 14 patients (82%) died due to aortic rupture, with a median survival time of 13 (10.5, 30.25) h. The causes of death in the remaining three patients were cerebral infarction, myocardial infarction, and multiple organ dysfunction syndrome, respectively.
No significant differences were observed between the ruptured and non-ruptured groups in terms of age, sex distribution, body mass index, medical history, misdiagnosis, or the number of transfers. The detailed clinical presentations of patients with TAAD upon hospital admission are provided in Table 1.
Table 1
Variables | Overall (n=30) | Non-rupture (n=16) | Rupture (n=14) | P value |
---|---|---|---|---|
Age (years) | 62.9±14.6 | 65.1±14.5 | 60.4±14.8 | 0.94 |
BMI (kg/m2) | 22.5±3.3 | 22.9±3.8 | 21.99±2.7 | 0.15 |
Male | 20 (66.7) | 9 (56.3) | 11 (78.6) | 0.20 |
Stage | 0.41 | |||
Hyperacute | 24 (80.0) | 12 (75.0) | 12 (85.7) | |
Acute | 4 (13.3) | 3 (18.8) | 1 (7.1) | |
Subacute | 1 (3.3) | 0 (0.0) | 1 (7.1) | |
Chronic | 1 (3.3) | 1 (6.3) | 0 (0.0) | |
Hypertension | 18 (60.0) | 10 (62.5) | 8 (57.1) | 0.77 |
Connective tissue disease | 3 (10.0) | 3 (18.8) | 0 (0.0) | 0.09 |
Misdiagnosed in other hospitals | 7 (23.3) | 3 (18.8) | 4 (28.6) | 0.53 |
Received anticoagulation therapy | 7 (23.3) | 5 (31.3) | 2 (14.3) | 0.27 |
Transfer times | 0.89 | |||
0 | 2 (6.7) | 1 (6.3) | 1 (7.1) | |
1 | 25 (83.3) | 13 (81.2) | 12 (85.7) | |
2 | 3 (10.0) | 2 (12.5) | 1 (7.1) | |
Shock | 6 (20.0) | 3 (18.8) | 3 (21.4) | 0.86 |
Pericardial effusion | 11 (36.7) | 5 (31.3) | 6 (42.9) | 0.51 |
Aortic regurgitation | 23 (76.7) | 11 (68.8) | 12 (85.7) | 0.27 |
Received endotracheal intubation | 7 (23.3) | 4 (25.0) | 3 (21.4) | 0.82 |
Pain requiring medication | 27 (90.0) | 14 (87.5) | 13 (92.9) | 0.63 |
Hypertension requiring medication | 20 (66.7) | 12 (75.0) | 8 (57.1) | 0.30 |
Data are presented as the mean ± standard deviation or n (%). BMI, body mass index.
The differences in morphological characteristics between the groups are presented in Table 2. In the analysis of the widest cross-sectional area, the FL radian was significantly higher in the rupture group [269° (255.50°, 285.75°)] than in the non-rupture group [250° (208.50°, 269.38°), P=0.04]. Additionally, the rupture group had a significantly longer FL AL (125.74±20.41 mm) than the non-rupture group (102.58±30.60 mm, P=0.02).
Table 2
Variables | Overall (n=30) | Non-rupture (n=16) | Rupture (n=14) | P value |
---|---|---|---|---|
Dmax (mm) | 51.4±9.38 | 49±8.48 | 54.14±9.90 | 0.14 |
FLL (mm) | 525 [447.75, 548.25] | 513 [471.25, 542.75] | 542 [441.5, 552.75] | 0.49 |
Dmax/FLL | 0.1 [0.09, 0.12] | 0.1 [0.09, 0.12] | 0.1 [0.09, 0.12] | 0.56 |
Radian (°) | 260.65 [238.75, 274.5] | 250 [208.50, 269.38] | 269 [255.50, 285.75] | 0.04 |
Radian ≥245° | 20 (66.7) | 8 (50.0) | 12 (85.7) | 0.045 |
AL (mm) | 113.39±28.45 | 102.58±30.60 | 125.74±20.41 | 0.02 |
AL ≥130 mm | 11 (36.7) | 3 (18.8) | 8 (57.1) | 0.03 |
AL/FLL | 0.23 [0.2, 0.27] | 0.21 [0.18, 0.27] | 0.24 [0.21, 0.29] | 0.18 |
Number of drainage arteries and tears | 16.97±9.21 | 18.56±10.75 | 15.14±7.00 | 0.32 |
Centerline distance1 (mm) | 93.79±22.21 | 92.41±20.64 | 95.36±24.57 | 0.72 |
Centerline distance2 (mm) | 126.83±22.48 | 125.81±20.95 | 128±24.87 | 0.80 |
Centerline distance3 (mm) | 391 [362, 417.75] | 374 [362, 394] | 416 [382.75, 435.25] | 0.02 |
Centerline distance3 ≥391 mm | 15 (50.0) | 4 (25.0) | 11 (78.6) | 0.003 |
ATI1 | 1.34±0.22 | 1.36±0.19 | 1.32±0.26 | 0.71 |
ATI2 | 1.34±0.19 | 1.37±0.19 | 1.29±0.19 | 0.22 |
ATI3 | 3.02 [2.67, 3.27] | 2.97 [2.42, 3.24] | 3.08 [2.69, 3.32] | 0.34 |
Dmax/height (mm/m) | 30.94±5.49 | 29.92±5.34 | 32.12±5.61 | 0.28 |
AL/height (mm/m) | 68.2±17.03 | 62.54±18.84 | 74.66±12.37 | 0.050 |
Centerline distance3/height (mm/m) | 237.67 [215.44, 247.86] | 231.29 [217.35, 236] | 248.13 [216.9, 265.67] | 0.14 |
Data are presented as mean ± standard deviation, median [interquartile range] or n (%). AL, the arc length of the false lumen; ATI, aortic tortuosity index; ATI1, aortic tortuosity index between sinus tube juncture to the opening of the innominate artery; ATI2, aortic tortuosity index between sinus tube juncture to the end of the left subclavian artery; ATI3, aortic tortuosity index between sinus tube juncture to the opening of the celiac trunk; Centerline distance1, centerline distance between sinus tube juncture to the opening of the innominate artery; Centerline distance2, centerline distance between sinus tube juncture to the end of left subclavian artery; Centerline distance3, centerline distance between sinus tube juncture to the opening of celiac trunk; CT, computed tomography; Dmax, the maximum diameter of the aorta; FL, false lumen; FLL, the length of the false lumen; LSA, left subclavian artery; Number of drainage arteries and tears, the number of intimal tears within the aorta and the number of branch arteries arising from false lumen; Radian, the curvature of the false lumen; STJ, sinus tube juncture.
No significant differences were observed between the rupture and non-rupture groups in terms of Dmax, FLL, Dmax/FLL ratio, AL/FLL ratio, the number of drainage arteries and tears, or the ATI across the three aortic segments and the ratio of Dmax, AL, and centerline distance between sinus tube juncture to the opening of celiac trunk (centerline distance3) to height. However, the centerline distance between the sinotubular junction (STJ) and centerline distance3 was significantly greater in the rupture group [416 (382.75, 435.25) mm] compared to the non-rupture group [374 (362, 394) mm, P=0.02]. No significant differences were found between the two groups in ATI for the third aortic segment (ATI3).
Single factor logistic regression analysis was conducted to identify significant variables associated with aortic rupture in patients with TAAD based on the factors that showed significant differences (Table 3). Two risk factors were identified: an FL AL of ≥130 mm (OR =5.78; 95% CI: 1.12–29.85; P=0.04) and centerline distance3 ≥391 mm (OR =11; 95% CI: 2–60.57; P=0.006).
Table 3
Variables | B | Standard error | Wald | Odds ratio | 95% confidence interval | P |
---|---|---|---|---|---|---|
Arc length ≥130 mm | 1.75 | 0.84 | 4.38 | 5.78 | 1.12–29.85 | 0.04 |
Centerline distance3 ≥391 mm | 2.40 | 0.87 | 7.59 | 11 | 2–60.57 | 0.006 |
Odds ratios and 95% confidence intervals were calculated by single factor logistic regression.
Discussion
Key findings
The incidence of TAAD is influenced by factors such as climate change and temporal variations, often resulting in the rapid admission of a large number of patients within a brief period (8,9). However, the treatment of TAAD necessitates complex and time-consuming aortic repair procedures, which must be conducted by specialized cardiothoracic surgical teams—a resource that is often scarce in our country. Consequently, some patients may not receive timely surgical intervention despite being hospitalized without financial constraints. Furthermore, patients presenting with malperfusion syndromes—such as myocardial infarction, coma, intestinal ischemia, and other serious complications—could not avoid critical anti-physiological measures during surgical treatment (e.g., cardiopulmonary bypass, deep hypothermic circulatory arrest). Unfortunately, these interventions can exacerbate existing organ damage, leading to poor surgical outcomes. As a result, some medical centers opt to delay surgery until the affected organ’s function has improved, at which point surgical intervention may be reconsidered. Such delays inevitably heighten the risk of aortic rupture during the waiting period.
This clinical dilemma necessitates that physicians evaluate patients who are at greater risk of aortic rupture, carefully weigh the benefits and risks, and allocate resources judiciously. Nonetheless, current risk assessment methodologies must be further refined to identify more valuable and sensitive indicators, particularly those related to imaging features, which have not garnered much attention to date. In this study, we identified two easily accessible morphological features from CTA during the initial hospitalization that are independently associated with aortic rupture in patients with TAAD.
Explanations of findings
Regarding the aneurysmal ascending aorta, the aortic diameter remains the primary metric upon which guidelines for aortic surveillance and intervention are based on (10). Wu et al. (6) found that a larger aortic diameter was correlated with a higher incidence of aortic rupture in patients with TAAD, positing that a greater aortic diameter indicates increased pressure and a thinner arterial wall. It has also been suggested that AHI provides a stronger predictive value for in-hospital rupture than the original aortic size (8). However, in our study, the Dmax and AHI did not demonstrate significant differences between the two groups.
The first morphological parameter related to aortic rupture identified in our patients was the FL radian, initially referred to as the circumferential extent of FL detachment by Sailer et al. (11). This novel parameter captures the physiology of dissection by quantifying the proportion of the weakened outer aortic wall exposed to FL pressure versus the proportion of the preserved outer aortic wall circumference exposed to true lumen pressure. A greater FL radian suggests a more extensive dissection of the aortic wall, thereby reflecting the extent of the dissection in the same orthogonal cross-section more directly than the Dmax and AHI do. Although the significance of the radian in the logistic regression analysis may be influenced by our small sample size, it highlights the potential utility of FL radian in predicting aortic rupture.
The second parameter, which is analogous to the FL radian, is FL AL, representing the degree of dissection at the maximum cross-section of the aorta in a similar manner. Given the complex and diverse anatomical changes associated with TAAD, we introduced this parameter specifically for aortas with irregular cross-sections. This index is more convenient to measure and offers greater accuracy in describing the extent of lesions compared to the FL radian. In our study, an AL of ≥130 mm was significantly associated with a 5.78-fold increased risk of ruptured TAAD. Therefore, an elevation in AL suggests a greater severity of aortic dissection and instability of the aortic wall, which correlates with in-hospital aortic rupture if the patient does not receive timely surgical intervention.
Recently, the length of the aorta has gained attention as a parameter worth analyzing in identifying patients at risk for adverse aortic events (AAEs) (10). An early investigation into the correlation between ascending aortic length (AAL) and AAEs was conducted by Krüger et al. (12), who demonstrated that classical AAL (c-AAL)—the distance between the STJ and brachiocephalic trunk—was significantly greater in patients with TAAD compared to healthy controls (108 vs. 84 mm, P>0.001). A risk-adjusted confirmation of these findings was provided by Heuts et al. (13), who, after propensity matching, found that c-AAL was significantly longer in patients with TAAD than in controls (78.6±8.8 vs. 68.9±7.2 mm, P<0.001). Notably, c-AAL independently predicted TAAD in multivariable regression analysis. Furthermore, surrogates of increased aortic length, such as a high ATI and a type II aorta, have also been linked to a higher rate of AAEs, both before and after aortic intervention. In a cohort of patients with Marfan syndrome followed for 4 years, those who developed dissection or underwent surgery exhibited a greater ATI at the baseline (14). Inspired by these findings, we measured the length and tortuosity of different aortic segments and identified a third significant parameter—the centerline distance (centerline3). Our results showed that this distance was significantly greater in the ruptured group than in the non-ruptured group [416 (382.75, 435.25) vs. 374 (362, 394) mm, P<0.05]. Furthermore, a centerline3 measurement of ≥391 mm was associated with an 11-fold increased risk of ruptured TAAD. This suggests that aortic elongation is part of a gradual remodeling process resulting from “material fatigue” of the vessel wall. Prolonged exposure to pulsatile strain can lead to the fracture and breakdown of elastin fibers, contributing to structural remodeling. In addition to elongation and dilation, this theory also accounts for age-related vascular stiffening, as the reduction in elastin content impairs vessel compliance (15,16). Such pathophysiological processes diminish aortic compliance, making the association between elongation and aortic rupture highly plausible.
Insufficient outflow, or a mismatch between inflow and outflow, can lead to increased pressure in FL. This may result in true lumen compression in the acute setting or FL expansion and aneurysm formation over time. Experimental and clinical studies have demonstrated that pulsatile inflow into a lumen with impaired outflow can significantly increase mean arterial and diastolic pressures compared to a lumen with adequate outflow, even when systolic pressures remain similar (17-21). Sailer et al. (11) found that greater drainage of the FL through large unobstructed branch arteries arising from the FL decreases the risk of adverse events. Each intercostal artery originating from FL may also create a small natural fenestration, facilitating outflow, even if such communications are too small to be detected with current CT technology during the acute phase. Building on these insights, we counted the number of intimal tears within the aorta and the number of branch arteries arising from FL, comprising the innominate, left common carotid, left subclavian, and intercostal arteries. We regarded the sum of these two metrics as the total number of FL outflow channels. We hypothesize that the presence of any exit from the FL can facilitate blood shunting, thereby reducing pressure within it. Although our analysis revealed no significant difference in the number of FL outflow channels between the ruptured group (15.14±7.00) and the non-ruptured group (18.56±10.75), this anatomical parameter may still indirectly reflect the hemodynamic status of FL. Its potential role in predicting aortic rupture warrants further exploration in future large-sample studies.
Strengths and limitations
As a single centered retrospective analysis, there are inherent limitations and biases in this study, including the relatively fewer cases, missing imaging data, and the lack of discharged patient’s records. Nonetheless, we propose that an imaging strategy that incorporates diverse anatomical factors could substantially improve existing risk assessment methods for predicting aortic rupture in patients with TAAD.
Conclusions
CT imaging morphological features in patients with TAAD demonstrated a strong correlation with the risk of aortic rupture. These features may serve as valuable predictors of individual rupture risk in internally validated models, thereby enabling timely surgical interventions for those at the highest risk.
Acknowledgments
None.
Footnote
Reporting Checklist: The authors have completed the STROBE reporting checklist. Available at https://jtd.amegroups.com/article/view/10.21037/jtd-24-1702/rc
Data Sharing Statement: Available at https://jtd.amegroups.com/article/view/10.21037/jtd-24-1702/dss
Peer Review File: Available at https://jtd.amegroups.com/article/view/10.21037/jtd-24-1702/prf
Funding: This work was sponsored by the guiding projects of
Conflicts of Interest: All authors have completed the ICMJE uniform disclosure form (available at https://jtd.amegroups.com/article/view/10.21037/jtd-24-1702/coif). The authors have no conflicts of interest to declare.
Ethical Statement: The authors are accountable for all aspects of the work in ensuring that questions related to the accuracy or integrity of any part of the work are appropriately investigated and resolved. The study was conducted in accordance with the Declaration of Helsinki and its subsequent amendments. The study protocol was approved by the Ethics Committee of Xiamen Cardiovascular Hospital (No. 2023036) and informed consent was taken from all the patients or their families.
Open Access Statement: This is an Open Access article distributed in accordance with the Creative Commons Attribution-NonCommercial-NoDerivs 4.0 International License (CC BY-NC-ND 4.0), which permits the non-commercial replication and distribution of the article with the strict proviso that no changes or edits are made and the original work is properly cited (including links to both the formal publication through the relevant DOI and the license). See: https://creativecommons.org/licenses/by-nc-nd/4.0/.
References
- Wang X, Ghayesh MH, Kotousov A, et al. Fluid-structure interaction study for biomechanics and risk factors in Stanford type A aortic dissection. Int J Numer Method Biomed Eng 2023;39:e3736. [Crossref] [PubMed]
- Harris KM, Nienaber CA, Peterson MD, et al. Early Mortality in Type A Acute Aortic Dissection: Insights From the International Registry of Acute Aortic Dissection. JAMA Cardiol 2022;7:1009-15. [Crossref] [PubMed]
- Inoue Y, Matsuda H, Uchida K, et al. Analysis of Acute Type A Aortic Dissection in Japan Registry of Aortic Dissection (JRAD). Ann Thorac Surg 2020;110:790-8. [Crossref] [PubMed]
- Li ZD, Liu Y, Zhu J, et al. Risk factors of pre-operational aortic rupture in acute and subacute Stanford type A aortic dissection patients. J Thorac Dis 2017;9:4979-87. [Crossref] [PubMed]
- Tang X, Jiang Y, Xue Y, et al. Predictive Factors for In-Hospital Preoperative Rupture in Hyperacute Type A Aortic Dissection. Heart Surg Forum 2021;24:E379-86. [Crossref] [PubMed]
- Wu J, Qiu J, Xie E, et al. Predicting in-hospital rupture of type A aortic dissection using Random Forest. J Thorac Dis 2019;11:4634-46. [Crossref] [PubMed]
- Booher AM, Isselbacher EM, Nienaber CA, et al. The IRAD classification system for characterizing survival after aortic dissection. Am J Med 2013;126:730.e19-24. [Crossref] [PubMed]
- Benouaich V, Soler P, Gourraud PA, et al. Impact of meteorological conditions on the occurrence of acute type A aortic dissections. Interact Cardiovasc Thorac Surg 2010;10:403-6. [Crossref] [PubMed]
- Takagi H, Ando T, Umemoto T, et al. Meta-Analysis of Seasonal Incidence of Aortic Dissection. Am J Cardiol 2017;120:700-7. [Crossref] [PubMed]
- Girardi LN, Lau C, Gambardella I. Aortic dimensions as predictors of adverse events. J Thorac Cardiovasc Surg 2021;161:1193-7. [Crossref] [PubMed]
- Sailer AM, van Kuijk SM, Nelemans PJ, et al. Computed Tomography Imaging Features in Acute Uncomplicated Stanford Type-B Aortic Dissection Predict Late Adverse Events. Circ Cardiovasc Imaging 2017;10:e005709. [Crossref] [PubMed]
- Krüger T, Sandoval Boburg R, Lescan M, et al. Aortic elongation in aortic aneurysm and dissection: the Tübingen Aortic Pathoanatomy (TAIPAN) project. Eur J Cardiothorac Surg 2018;54:26-33. [Crossref] [PubMed]
- Heuts S, Adriaans BP, Gerretsen S, et al. Aortic elongation part II: the risk of acute type A aortic dissection. Heart 2018;104:1778-82. [Crossref] [PubMed]
- Franken R, El Morabit A, de Waard V, et al. Increased aortic tortuosity indicates a more severe aortic phenotype in adults with Marfan syndrome. Int J Cardiol 2015;194:7-12. [Crossref] [PubMed]
- Sokolis DP. Passive mechanical properties and structure of the aorta: segmental analysis. Acta Physiol (Oxf) 2007;190:277-89. [Crossref] [PubMed]
- Sokolis DP, Boudoulas H, Karayannacos PE. Segmental differences of aortic function and composition: clinical implications. Hellenic J Cardiol 2008;49:145-54.
- Winnerkvist A, Lockowandt U, Rasmussen E, et al. A prospective study of medically treated acute type B aortic dissection. Eur J Vasc Endovasc Surg 2006;32:349-55. [Crossref] [PubMed]
- Berguer R, Parodi JC, Schlicht M, et al. Experimental and clinical evidence supporting septectomy in the primary treatment of acute type B thoracic aortic dissection. Ann Vasc Surg 2015;29:167-73. [Crossref] [PubMed]
- Chung JW, Elkins C, Sakai T, et al. True-lumen collapse in aortic dissection: part I. Evaluation of causative factors in phantoms with pulsatile flow. Radiology 2000;214:87-98. [Crossref] [PubMed]
- Williams DM, Lee DY, Hamilton BH, et al. The dissected aorta: part III. Anatomy and radiologic diagnosis of branch-vessel compromise. Radiology 1997;203:37-44. [Crossref] [PubMed]
- Parodi JC, Berguer R, Ferreira LM, et al. Intra-aneurysmal pressure after incomplete endovascular exclusion. J Vasc Surg 2001;34:909-14. [Crossref] [PubMed]